Calculate the Bond Order of CO: A Comprehensive Guide
Bond order, a critical concept in chemistry, provides insights into the strength and nature of chemical bonds. Understanding how to find the bond order of carbon monoxide (CO) is fundamental in various scientific disciplines. This article will delve into the definition, importance, and historical significance of bond order, guiding readers through the steps to accurately calculate the bond order of CO.
Bond Order of Carbon Monoxide
Understanding the bond order of carbon monoxide (CO) is critical in chemistry as it provides insights into the molecule’s properties and reactivity. The bond order is a measure of the strength and nature of the bond between the carbon and oxygen atoms.
- Molecular Orbital Theory
- Electron Configuration
- Bond Length
- Bond Strength
- Magnetic Properties
- Chemical Reactivity
- Spectroscopic Properties
- Thermodynamic Stability
- Applications in Catalysis
- Environmental Implications
These aspects are interconnected and provide a comprehensive understanding of the bond order of CO. By exploring these aspects, chemists can gain valuable insights into the behavior and applications of CO in various chemical systems.
Molecular Orbital Theory
Molecular Orbital Theory (MOT) provides a theoretical framework for understanding the electronic structure and bonding in molecules. In the context of determining the bond order of carbon monoxide (CO), MOT offers valuable insights into the nature of the chemical bond.
- Linear Combination of Atomic Orbitals (LCAO): MOT describes molecular orbitals as a combination of atomic orbitals, providing a qualitative understanding of the bonding interactions in CO.
- Symmetry and Overlap: The symmetry and overlap of atomic orbitals determine the shape and energy of molecular orbitals, influencing the bond order and properties of CO.
- aufbau Principle and Hund’s Rule: These principles govern the filling of molecular orbitals with electrons, dictating the electronic configuration and bond order of CO.
- Molecular Orbital Diagrams: Visual representations of molecular orbitals help visualize the distribution of electrons and predict the bond order and reactivity of CO.
By considering these facets of MOT, chemists can gain a deeper understanding of the electronic structure and bonding in CO, enabling more accurate calculations of bond order and predictions of its chemical behavior.
Electron Configuration
Understanding the electron configuration of carbon monoxide (CO) is crucial for determining its bond order. Electron configuration refers to the distribution of electrons in atomic orbitals, providing insights into the chemical properties and bonding behavior of CO.
- Atomic Orbitals: Electrons occupy specific atomic orbitals, which are characterized by their energy levels and shapes. In CO, the carbon atom has six valence electrons, while the oxygen atom has four.
- Aufbau Principle: Electrons fill atomic orbitals in order of increasing energy, following the Aufbau principle. The Aufbau diagram for CO shows the distribution of electrons in its atomic orbitals.
- Hund’s Rule: Hund’s rule states that electrons occupy degenerate orbitals (orbitals with the same energy) with parallel spins before pairing. This rule influences the electron configuration of CO.
- Molecular Orbital Formation: The atomic orbitals of carbon and oxygen overlap to form molecular orbitals, which are new orbitals that describe the electron distribution in the CO molecule.
By considering these aspects of electron configuration, chemists can gain insights into the electronic structure of CO, enabling more accurate calculations of bond order and predictions of its chemical behavior.
Bond Length
Bond length, a crucial parameter in chemistry, provides valuable insights into the nature and stability of chemical bonds. In the context of determining the bond order of carbon monoxide (CO), bond length plays a significant role.
Bond length is inversely related to bond order. A shorter bond length typically indicates a higher bond order, reflecting a stronger bond between the atoms. Conversely, a longer bond length corresponds to a lower bond order, indicating a weaker bond. This relationship arises from the interplay between bond strength and bond order. Stronger bonds, characterized by higher bond orders, have shorter bond lengths due to the increased electron density and attraction between the bonded atoms.
In the case of CO, the bond length is a critical component of determining the bond order. Experimental measurements or computational methods can be used to determine the bond length, which then provides valuable information about the strength and nature of the carbon-oxygen bond. Understanding the bond length enables chemists to make inferences about the electronic structure, reactivity, and spectroscopic properties of CO.
Bond Strength
Bond strength, an essential aspect of “how to find the bond order of co,” provides crucial insights into the stability and reactivity of the carbon monoxide molecule. It is directly related to the bond order, with stronger bonds typically having higher bond orders. Several factors contribute to bond strength, each playing a role in determining the overall strength of the carbon-oxygen bond in CO.
- Bond Dissociation Energy: The energy required to break a bond, providing a quantitative measure of bond strength. A higher bond dissociation energy indicates a stronger bond.
- Bond Length: Inversely related to bond strength, bond length measures the distance between bonded atoms. Shorter bond lengths generally correspond to stronger bonds due to increased electron density and attraction.
- Bond Order: Directly proportional to bond strength, bond order reflects the number of electron pairs shared between bonded atoms. Higher bond orders indicate stronger bonds.
- Electronegativity: The ability of atoms to attract electrons, influencing bond strength. Bonds between atoms with similar electronegativities tend to be stronger due to more balanced electron distribution.
Understanding these components of bond strength allows chemists to accurately determine the bond order of CO and gain insights into its chemical properties and behavior. Bond strength is a fundamental concept in chemistry, providing a deeper understanding of molecular stability, reactivity, and various chemical phenomena.
Magnetic Properties
Magnetic properties play a crucial role in understanding “how to find the bond order of co”. The bond order, which measures the strength and nature of the chemical bond between carbon and oxygen in CO, can be influenced by the magnetic properties of the molecule.
The magnetic properties of CO arise from the presence of unpaired electrons. In a molecule with unpaired electrons, the electrons have parallel spins, creating a magnetic field. The strength of the magnetic field is directly related to the number of unpaired electrons. In the case of CO, there are two unpaired electrons, resulting in a paramagnetic molecule.
The magnetic properties of CO can be used to determine the bond order. Paramagnetic molecules have higher bond orders than diamagnetic molecules. This is because the unpaired electrons in paramagnetic molecules contribute to the overall bond strength. Therefore, by measuring the magnetic properties of CO, chemists can gain insights into the bond order of the molecule.
In summary, understanding the magnetic properties of CO is a critical component of “how to find the bond order of co”. The magnetic properties provide valuable information about the number of unpaired electrons and the strength of the bond between carbon and oxygen. This understanding has practical applications in various fields of science, including chemistry, materials science, and catalysis.
Chemical Reactivity
Chemical reactivity, a central aspect of “how to find the bond order of co,” encompasses the propensity of a molecule to undergo chemical transformations. Understanding this reactivity provides insights into the behavior and applications of CO in various chemical systems.
- Bond Strength and Reactivity: Bond order, a measure of bond strength, influences chemical reactivity. Stronger bonds (higher bond orders) tend to be less reactive, while weaker bonds (lower bond orders) are more reactive.
- Reaction Pathways: The bond order of CO affects the reaction pathways available to the molecule. Different bond orders can lead to distinct reaction intermediates and products.
- Catalysis: CO’s bond order modulates its ability to act as a catalyst in chemical reactions. The bond order influences the molecule’s adsorption and activation properties on catalyst surfaces.
- Environmental Implications: CO’s chemical reactivity plays a role in its environmental impact. The bond order affects the molecule’s stability in the atmosphere and its interactions with other species, influencing its contribution to air pollution and climate change.
In summary, chemical reactivity is a multifaceted aspect of “how to find the bond order of co” that provides insights into the behavior and applications of CO in chemistry. Understanding the bond order’s influence on reactivity enables chemists to predict and control chemical reactions involving CO, contributing to advancements in catalysis, environmental science, and various other fields.
Spectroscopic Properties
Spectroscopic Properties play a crucial role in the determination of “how to find the bond order of co”. Spectroscopy is a technique that analyzes the interaction of electromagnetic radiation with matter. It provides insights into the energy levels, molecular structure, and bonding of molecules.
The bond order of CO can be determined by analyzing its spectroscopic properties, such as its vibrational and electronic spectra. The vibrational spectrum of CO exhibits a characteristic peak that corresponds to the stretching vibration of the carbon-oxygen bond. The wavenumber of this peak is directly related to the bond order. A higher bond order results in a higher wavenumber. Similarly, the electronic spectrum of CO can provide information about the energy difference between different molecular orbitals, which is influenced by the bond order.
Understanding the relationship between spectroscopic properties and bond order has practical applications in various fields. For example, in environmental monitoring, spectroscopic techniques are used to measure the concentration of CO in the atmosphere. In industrial settings, spectroscopy is employed to optimize chemical processes involving CO. Additionally, spectroscopic studies contribute to the development of new materials and catalysts that utilize CO.
In summary, spectroscopic properties are essential for understanding “how to find the bond order of co”. By analyzing the spectroscopic data, chemists can gain insights into the bonding and electronic structure of CO, enabling them to predict and control its behavior in chemical reactions and applications.
Thermodynamic Stability
Thermodynamic stability plays a critical role in determining the “how to find the bond order of co”. It refers to the tendency of a molecule to maintain its structural integrity and resist change under specific conditions. Understanding the thermodynamic stability of CO provides insights into its behavior in various chemical reactions and applications.
- Bond Strength: The strength of the carbon-oxygen bond directly influences the thermodynamic stability of CO. A higher bond order, indicating a stronger bond, leads to increased stability.
- Molecular Geometry: The linear geometry of CO contributes to its stability by minimizing steric hindrance and maximizing bond strength.
- Electronic Structure: The electronic configuration of CO, with its filled valence orbitals, promotes stability by reducing its reactivity.
- Environmental Factors: External factors such as temperature and pressure can affect the thermodynamic stability of CO. Higher temperatures can weaken the bond, while increased pressure can favor the formation of CO.
In summary, the thermodynamic stability of CO is governed by the interplay of bond strength, molecular geometry, electronic structure, and environmental factors. Understanding these aspects enables chemists to predict the behavior of CO in different conditions and optimize its use in various chemical processes and applications.
Applications in Catalysis
The understanding of bond order in carbon monoxide (CO) plays a pivotal role in its applications in catalysis. Catalysis involves the use of a catalyst, a substance that enhances the rate of a chemical reaction without being consumed. By manipulating the bond order of CO, chemists can tailor its catalytic activity and selectivity for specific reactions.
One prominent example lies in the field of heterogeneous catalysis, where CO serves as a key intermediate in various industrial processes. The bond order of CO influences its adsorption and activation properties on catalyst surfaces. By optimizing the bond order, researchers can design catalysts with enhanced activity and selectivity for reactions such as the Fischer-Tropsch process, which converts CO and hydrogen into liquid fuels.
Furthermore, understanding the bond order of CO is crucial in the development of homogeneous catalysts, where CO acts as a ligand that binds to metal ions. The bond order between CO and the metal center directly affects the electronic structure and reactivity of the catalyst. By fine-tuning the bond order, chemists can modulate the catalyst’s performance for specific reactions, such as carbonylation reactions, which involve the insertion of CO into organic molecules.
In summary, the study of bond order in CO provides a foundation for understanding its applications in catalysis. By manipulating the bond order, researchers can design catalysts with tailored activity and selectivity for a wide range of chemical reactions, leading to advancements in various industries and technologies.
Environmental Implications
Understanding the bond order of carbon monoxide (CO) is crucial for assessing its environmental implications. CO is a colorless, odorless, and toxic gas that contributes to air pollution and climate change. Its bond order influences its stability, reactivity, and interactions with other molecules in the environment.
The bond order of CO affects its atmospheric lifetime. A higher bond order leads to a more stable CO molecule, which persists longer in the atmosphere. This prolonged presence contributes to the greenhouse effect, as CO traps heat and contributes to global warming. Additionally, CO’s bond order influences its reactivity with other atmospheric species, including hydroxyl radicals (OH) and nitrogen oxides (NOx). These reactions play a role in the formation of tropospheric ozone, a harmful air pollutant that can cause respiratory problems and damage vegetation.
Furthermore, the bond order of CO is relevant in the context of industrial processes and combustion engines. Incomplete combustion in these processes can lead to the release of CO into the environment. Understanding the bond order of CO provides insights into its behavior and allows researchers to develop strategies for reducing CO emissions. For example, optimizing combustion conditions and employing catalytic converters can help minimize CO formation and mitigate its environmental impact.
In summary, the bond order of CO is a critical component for understanding its environmental implications. It influences the stability, reactivity, and atmospheric lifetime of CO, affecting its contribution to air pollution and climate change. By comprehending the bond order of CO, scientists and policymakers can develop informed approaches to mitigate its environmental impact and protect human health and ecosystems.
Frequently Asked Questions About How to Find the Bond Order of CO
This section aims to address common questions and provide concise answers to help clarify different aspects of “how to find the bond order of co”.
Question 1: Why is it crucial to determine the bond order of CO?
Answer: Determining the bond order of CO is crucial for understanding its molecular structure, stability, reactivity, and magnetic properties. It also provides insights into CO’s behavior in various chemical processes and its environmental implications.
Question 6: How does the bond order of CO affect its environmental impact?
Answer: The bond order of CO influences its atmospheric lifetime, reactivity, and interactions with other molecules in the environment. This affects its contribution to air pollution, climate change, and the formation of harmful substances like tropospheric ozone.
In summary, understanding the bond order of CO is essential for grasping its fundamental properties and behavior. These FAQs provide a deeper understanding of the significance and applications of bond order in CO, paving the way for further exploration of its chemistry and its role in various scientific disciplines.
The following section will delve into the practical methods and techniques used to determine the bond order of CO, enabling readers to apply this knowledge in their own research and understanding.
Tips for Determining Bond Order
Understanding the nuances of determining bond order is crucial for comprehending molecular structure and reactivity. Here are some practical tips to enhance your understanding and application of this concept:
Tip 1: Master Molecular Orbital Theory: Delve into molecular orbital theory to grasp the electronic structure and bonding interactions that govern bond order.
Tip 2: Analyze Electron Configuration: Study the distribution of electrons in atomic orbitals to determine the number of bonding and anti-bonding electrons, which directly influences bond order.
Tip 3: Measure Bond Length: Utilize experimental techniques or computational methods to determine the distance between bonded atoms. Shorter bond lengths often indicate higher bond orders.
Tip 4: Assess Bond Strength: Explore the energy required to break a bond, which provides insights into bond order and the stability of the molecule.
Tip 5: Examine Magnetic Properties: Analyze the magnetic behavior of a molecule to determine the presence of unpaired electrons, which can influence bond order.
Tip 6: Study Chemical Reactivity: Investigate how bond order affects the reactivity of a molecule, as stronger bonds tend to be less reactive.
Tip 7: Analyze Spectroscopic Data: Utilize spectroscopic techniques to gather information about vibrational and electronic transitions, which can provide insights into bond order.
Tip 8: Consider Thermodynamic Stability: Understand the factors that influence the stability of a molecule, including bond order, molecular geometry, and electronic structure.
These tips will empower you to confidently determine bond order and gain valuable insights into molecular properties and behavior. By applying these principles, you can expand your understanding of chemical bonding and its implications in various scientific disciplines.
In the concluding section, we will explore advanced applications of bond order determination, highlighting its significance in fields such as catalysis, environmental chemistry, and materials science.
Conclusion
This article has provided a comprehensive exploration of “how to find the bond order of co”. By understanding the underlying principles and applying the discussed techniques, scientists and researchers can accurately determine bond order and gain valuable insights into the molecular properties and behavior of carbon monoxide.
Throughout the article, we have emphasized the significance of molecular orbital theory, electron configuration, and experimental measurements in determining bond order. These concepts are interconnected and provide a holistic approach to understanding the nature of chemical bonds. Additionally, we have explored the practical applications of bond order determination in fields such as catalysis, environmental chemistry, and materials science, highlighting its impact on various scientific disciplines.
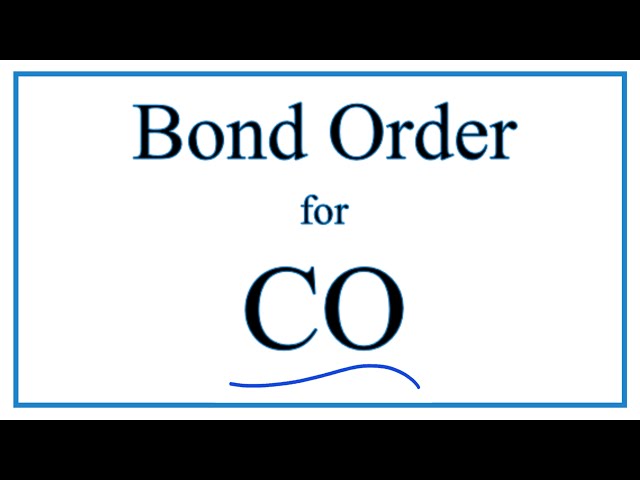