Bond order, a crucial concept in chemistry, measures the strength of a chemical bond. It is defined as the number of electron pairs shared between two atoms and is a key indicator of bond properties. For instance, a double bond, such as the one in oxygen (O2), has a bond order of two due to the sharing of two electron pairs between the oxygen atoms. Understanding how to calculate bond order is essential for comprehending molecular structure and reactivity.
Calculating bond order provides valuable insights into the nature of chemical bonds. It helps predict bond length, strength, and reactivity. Historically, Linus Pauling developed the concept of bond order in the 1930s as part of his pioneering work on quantum chemistry.
In this article, we will delve into the methods for calculating bond order, exploring the factors that influence it and its applications in understanding chemical bonding.
How to Calculate Bond Order in Chemistry
Calculating bond order is crucial for understanding the strength and properties of chemical bonds. Here are 10 key aspects to consider:
- Number of shared electrons
- Bond length
- Bond strength
- Molecular orbital theory
- Resonance structures
- Hybridization
- Electronegativity
- Lewis structures
- Quantum chemistry
- Periodic trends
These aspects are interconnected and provide a comprehensive understanding of bond order. For example, the number of shared electrons determines the bond order, which in turn influences bond length and strength. Molecular orbital theory explains the electronic structure of molecules and provides a deeper understanding of bond formation. Resonance structures and hybridization help explain the behavior of complex molecules with multiple bonds. Electronegativity and periodic trends offer insights into the reactivity and properties of different elements and their bonds.
Number of shared electrons
The number of shared electrons between two atoms is a critical component of bond order calculation. Bond order, a measure of the strength and type of chemical bond, is directly proportional to the number of shared electrons. A single bond represents the sharing of one electron pair, a double bond involves two shared electron pairs, and so on. For instance, in the oxygen molecule (O2), each oxygen atom contributes one electron to form a double bond, resulting in a bond order of two.
Understanding the relationship between the number of shared electrons and bond order helps predict the properties and reactivity of molecules. For example, molecules with higher bond orders tend to be shorter and stronger. This knowledge is essential in various fields such as materials science, catalysis, and drug design.
In conclusion, the number of shared electrons plays a fundamental role in determining bond order, offering insights into the nature and behavior of chemical bonds. This understanding underpins numerous applications in diverse scientific disciplines.
Bond length
In the context of bond order calculation, bond length, defined as the distance between the nuclei of two bonded atoms, plays a crucial role in understanding bond strength and properties. Several factors influence and are influenced by bond length, providing valuable insights into the nature of chemical bonds.
- Atomic radii
The size of the atoms involved directly affects bond length. Larger atoms generally lead to longer bonds due to increased internuclear distance. - Bond order
Bond order and bond length have an inverse relationship. Higher bond orders correspond to shorter bond lengths due to stronger attractive forces between the nuclei. - Hybridization
The hybridization of atomic orbitals influences bond length by determining the shape and overlap of electron orbitals involved in bonding. Different hybridization types result in different bond lengths. - Electronegativity
Electronegativity, a measure of an atom’s ability to attract electrons, affects bond length. Bonds between atoms with significant electronegativity differences tend to be shorter and stronger.
Understanding the relationship between bond length and these factors enables chemists to predict bond properties, design new materials with tailored properties, and gain insights into the behavior of molecules in various chemical reactions.
Bond strength
Bond strength, a measure of the energy required to break a chemical bond, is intricately connected to bond order calculation in chemistry. Bond order, an indicator of the number of electron pairs shared between atoms, directly influences bond strength. Higher bond orders correspond to stronger bonds due to increased electron sharing and stronger attractive forces between the nuclei.
For instance, in the case of carbon-carbon bonds, a single bond has a bond order of one and a bond strength of approximately 347 kJ/mol. In contrast, a carbon-carbon double bond, with a bond order of two, exhibits a significantly higher bond strength of approximately 615 kJ/mol. This relationship is observed across various types of chemical bonds.
Understanding the connection between bond order and bond strength is crucial in various fields of chemistry. It enables the prediction of the stability and reactivity of molecules, the design of new materials with tailored properties, and the development of chemical processes that optimize bond formation and breaking.
Molecular orbital theory
Molecular orbital theory (MOT) is a fundamental concept in quantum chemistry that provides a mathematical framework to describe the electronic structure of molecules. It is a powerful tool for understanding and predicting chemical bonding and properties, including bond order calculation.
- Atomic orbitals
MOT starts by describing the atomic orbitals of individual atoms, which are mathematical functions that represent the wave-like behavior of electrons. These orbitals can be combined to form molecular orbitals, which describe the electron distribution in a molecule.
- Linear combination of atomic orbitals (LCAO)
LCAO is a technique used in MOT to construct molecular orbitals by combining atomic orbitals. The coefficients in the LCAO determine the extent to which each atomic orbital contributes to the molecular orbital.
- Molecular orbital energy levels
The energy of a molecular orbital is determined by the energy of the atomic orbitals that combine to form it. Molecular orbitals with lower energy are more stable and are typically occupied by electrons.
- Bond order
The bond order of a chemical bond is related to the number of electrons occupying the bonding molecular orbitals. Higher bond orders indicate stronger bonds.
In summary, molecular orbital theory provides a powerful framework for understanding and calculating bond order in chemistry. It enables chemists to predict the electronic structure, bonding, and properties of molecules, facilitating the design and development of new materials and technologies.
Resonance structures
Resonance structures, an important concept in chemistry, play a crucial role in calculating bond order and understanding the electronic structure of molecules. They provide a way to represent the delocalization of electrons in certain molecules, offering a more accurate description of their bonding than a single Lewis structure.
- Equivalent resonance structures
Resonance structures are different Lewis structures that represent the same molecule, differing only in the placement of electrons. They have the same number of covalent bonds and lone pairs, but the distribution of electrons is different.
- Average bond order
The bond order in a resonance structure is an average of the bond orders in all the contributing resonance structures. This concept is crucial for accurately predicting the strength and properties of chemical bonds.
- Delocalized electrons
Resonance structures indicate that certain electrons in a molecule are not confined to a specific bond but are delocalized over several atoms. This delocalization results in increased bond stability and affects the molecule’s overall properties.
- Benzene ring
A classic example of resonance is the benzene ring, which has six equivalent resonance structures. This resonance contributes to the stability and unique properties of benzene, such as its aromaticity.
In summary, resonance structures provide a deeper understanding of the electronic structure of molecules, allowing for more accurate bond order calculations. This understanding is essential in predicting the properties and reactivity of various chemical compounds.
Hybridization
Hybridization, a fundamental concept in chemistry, plays a pivotal role in calculating bond order and understanding the electronic structure of molecules. It describes the mixing of atomic orbitals to form new hybrid orbitals with different shapes and properties.
- sp Hybridization
In sp hybridization, one s orbital and one p orbital combine to form two equivalent sp hybrid orbitals. These hybrid orbitals are oriented 180 apart and form strong sigma bonds. A classic example is the linear geometry of BeCl2. - sp2 Hybridization
In sp2 hybridization, one s orbital and two p orbitals combine to form three equivalent sp2 hybrid orbitals. These hybrid orbitals are arranged in a trigonal planar geometry with 120 bond angles. An example is the trigonal planar structure of BF3. - sp3 Hybridization
In sp3 hybridization, one s orbital and three p orbitals combine to form four equivalent sp3 hybrid orbitals. These hybrid orbitals point towards the corners of a tetrahedron, forming strong sigma bonds. A well-known example is the tetrahedral geometry of methane (CH4). - dsp3 Hybridization
In dsp3 hybridization, one d orbital, one s orbital, and three p orbitals combine to form five equivalent dsp3 hybrid orbitals. These hybrid orbitals form octahedral complexes, as seen in SF6.
In summary, hybridization provides a deeper understanding of the electronic structure of molecules, enabling more accurate bond order calculations. The types of hybridization and their implications are crucial for predicting the geometry, bonding, and properties of various chemical compounds.
Electronegativity
Electronegativity, a crucial chemical property, plays a significant role in understanding and calculating bond order. It refers to the ability of an atom to attract electrons towards itself in a chemical bond. The electronegativity of an atom is influenced by factors such as its atomic number and atomic size.
Electronegativity directly impacts bond order because it determines the distribution of electrons in a chemical bond. Atoms with higher electronegativity tend to attract electrons more strongly, resulting in a higher electron density around them. This unequal distribution of electrons leads to the formation of polar bonds, where one atom has a partial positive charge and the other has a partial negative charge. The polarity of a bond affects its strength and reactivity.
For instance, in a bond between hydrogen and chlorine (HCl), chlorine is more electronegative than hydrogen. As a result, the electron density is shifted towards chlorine, creating a polar bond with a partial positive charge on hydrogen and a partial negative charge on chlorine. This polarity contributes to the strength and reactivity of the HCl bond.
Understanding the relationship between electronegativity and bond order is essential for various applications in chemistry. It helps predict the polarity of bonds, estimate bond strengths, and explain the behavior of molecules in chemical reactions. This knowledge is valuable in fields such as materials science, pharmaceutical development, and environmental chemistry, guiding the design and optimization of materials, drugs, and chemical processes.
Lewis structures
Lewis structures, a fundamental tool in chemistry, provide a simplified representation of the covalent bonding in molecules. They are crucial for understanding and calculating bond order, offering valuable insights into the electronic structure and properties of chemical compounds.
- Atoms and Bonds
Lewis structures depict atoms as circles with dots representing valence electrons. Lines connecting the circles represent covalent bonds, each formed by a shared pair of electrons. - Octet rule
Lewis structures are drawn to satisfy the octet rule, where atoms aim to have eight valence electrons, except for hydrogen, which aims for two. This rule helps predict the stability and bonding preferences of atoms. - Resonance structures
In some cases, multiple Lewis structures can be drawn for a molecule. These resonance structures represent different electron arrangements that contribute to the overall electronic structure. Resonance affects bond order calculations and provides a more accurate description of the molecule’s bonding. - Formal charges
Formal charges are assigned to atoms in Lewis structures to assess the distribution of electrons. These charges help identify polar bonds and predict the reactivity of the molecule.
In summary, Lewis structures provide a systematic way to represent and analyze the bonding in molecules, enabling the calculation of bond order. By understanding the components, implications, and applications of Lewis structures, chemists can gain insights into the electronic structure, bonding, and properties of various chemical compounds.
Quantum chemistry
Quantum chemistry plays a crucial role in understanding and calculating bond order in chemistry. It provides the theoretical framework that describes the electronic structure and bonding of atoms and molecules, enabling the prediction of their properties and behavior.
The fundamental principles of quantum mechanics, such as the wave-particle duality of electrons and the quantization of energy levels, are applied in quantum chemistry to determine the electronic wave functions of molecules. These wave functions describe the probability distribution of electrons within the molecule and are essential for calculating bond order.
One of the most important applications of quantum chemistry in calculating bond order is the Hartree-Fock method. This method approximates the wave function of a molecule as a product of one-electron wave functions and uses variational techniques to minimize the energy of the system. The resulting wave function can be used to calculate the electron density and bond order of the molecule.
Quantum chemistry is essential for understanding and calculating bond order in chemistry. It provides the theoretical foundation for predicting the properties and behavior of molecules and plays a vital role in various fields such as materials science, drug design, and computational chemistry.
Periodic trends
Periodic trends play a significant role in understanding and calculating bond order in chemistry. They refer to the patterns and regularities observed in the properties of elements as their atomic numbers increase. These trends provide valuable insights into the electronic structure and bonding behavior of atoms.
- Electronegativity
Electronegativity is a measure of an atom’s ability to attract electrons. It increases from left to right across a period and decreases down a group. Higher electronegativity leads to stronger bond polarity and influences bond order calculations.
- Atomic radius
Atomic radius is the distance from the nucleus to the outermost electron shell. It generally increases down a group and decreases across a period. Larger atomic radii result in weaker bonds and lower bond orders.
- Ionization energy
Ionization energy is the energy required to remove an electron from an atom. It increases from left to right across a period and decreases down a group. Higher ionization energies indicate stronger bonds and higher bond orders.
- Oxidation states
Oxidation states represent the charge of an atom in a compound. They vary depending on the element’s position in the periodic table and can influence bond order calculations, especially in transition metals and coordination complexes.
By considering periodic trends, chemists can gain a deeper understanding of the factors that influence bond order. These trends help predict bond strengths, explain chemical reactivity, and guide the design of new materials and compounds. They are essential in various fields, including inorganic chemistry, materials science, and catalysis.
FAQs on Calculating Bond Order in Chemistry
The following FAQs provide quick answers to common questions and clarify key aspects of bond order calculation in chemistry.
Question 1: What is bond order?
Bond order refers to the number of electron pairs shared between two atoms in a chemical bond, indicating the strength and type of bond.
Question 2: Why is calculating bond order important?
Bond order provides insights into bond strength, length, and reactivity, helping predict molecular properties and behavior.
Question 3: How do I calculate bond order using the molecular orbital theory?
In the molecular orbital theory, bond order is related to the number of electrons occupying bonding molecular orbitals.
Question 4: Can resonance affect bond order?
Yes, resonance structures contribute to bond order calculation by providing an average of bond orders over different resonance forms.
Question 5: How does electronegativity influence bond order?
Electronegativity affects bond polarity and electron distribution, which in turn influences bond order.
Question 6: Are there periodic trends in bond order?
Yes, periodic trends, such as electronegativity and atomic radius, can guide predictions of bond order and bond properties.
These FAQs offer a concise overview of bond order calculations in chemistry. For a more in-depth understanding of the concepts and applications, continue reading the following sections.
Moving forward, we will explore advanced techniques for calculating bond order, including quantum chemical methods and spectroscopic approaches.
Tips on Calculating Bond Order in Chemistry
Understanding and calculating bond order is crucial for comprehending the nature of chemical bonds. Here are some practical tips to enhance your understanding:
Tip 1: Focus on Shared Electrons
Bond order is directly related to the number of electron pairs shared between atoms.
Tip 2: Consider Electronegativity
Electronegativity influences electron distribution and bond polarity, affecting bond order.
Tip 3: Apply Resonance Structures
In resonance structures, the bond order is an average over all contributing structures.
Tip 4: Utilize Molecular Orbital Theory
Molecular orbital theory provides a framework to calculate bond order based on electron occupancy in bonding orbitals.
Tip 5: Understand Hybridization
Hybridization affects the geometry and bond order of molecules.
Tip 6: Employ Spectroscopic Techniques
Spectroscopic methods, such as IR and UV-Vis, can provide insights into bond order and molecular structure.
Tip 7: Use Computational Chemistry
Computational chemistry methods, like density functional theory, can accurately calculate bond order.
Tip 8: Relate to Periodic Trends
Periodic trends in electronegativity and atomic radius can guide predictions of bond order.
By following these tips, you can effectively calculate and analyze bond order, gaining a deeper understanding of chemical bonding and molecular properties.
In the next section, we will explore the applications of bond order calculations in various fields of chemistry.
Conclusion
In this comprehensive exploration of bond order calculation in chemistry, we have elucidated the fundamental principles, methods, and applications of this crucial concept. By understanding the number of shared electrons, considering electronegativity, utilizing resonance structures, and employing molecular orbital theory, chemists can effectively calculate bond order, gaining insights into bond strength, length, and reactivity.
The interconnectedness of these concepts highlights the complex nature of chemical bonding. Bond order is not merely a static value but rather a dynamic property influenced by various factors. This understanding empowers chemists to predict molecular properties, design new materials, and comprehend chemical reactions.
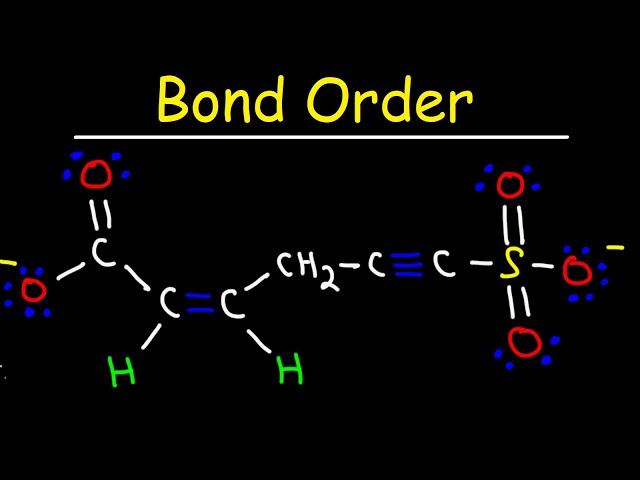