Bond order, a fundamental concept in chemistry, describes the strength and nature of the chemical bond between atoms. Understanding how to calculate bond order for a diatomic molecule like carbon dioxide (CO2) is essential for comprehending its molecular structure and properties.
Bond order provides valuable insights into the stability, reactivity, and electronic configuration of molecules. Historically, the concept of bond order was introduced by Linus Pauling in 1931, revolutionizing our understanding of chemical bonding.
In this article, we will delve into the intricacies of calculating bond order for CO2, exploring its significance and providing step-by-step instructions. By examining the distribution of electrons within the molecule, we gain a deeper understanding of its chemical nature and behavior.
How to Calculate Bond Order for CO2
Determining bond order is crucial for understanding the molecular structure and properties of CO2. Here are 10 key aspects to consider:
- Molecular Orbital Theory
- Valence Electrons
- Hybridization
- Resonance
- Bond Length
- Bond Strength
- Magnetic Properties
- Oxidation States
- Molecular Geometry
- Spectroscopic Properties
These aspects are interconnected and provide insights into the electronic structure, bonding, and behavior of CO2. Understanding bond order allows us to predict and explain a wide range of chemical phenomena, from molecular stability to reactivity.
Molecular Orbital Theory
Molecular Orbital Theory is a cornerstone of quantum chemistry, providing the theoretical framework for understanding the electronic structure of molecules, which is essential for calculating bond order. It posits that electrons occupy molecular orbitals, which are mathematical functions describing the electron’s wave-like properties within the molecule.
- Linear Combination of Atomic Orbitals (LCAO): The molecular orbitals are constructed by combining the atomic orbitals of the constituent atoms, leading to the formation of bonding and antibonding molecular orbitals.
- aufbau Principle: Electrons fill the molecular orbitals in order of increasing energy, following the same rules as atomic orbitals.
- Pauli Exclusion Principle: Each molecular orbital can accommodate a maximum of two electrons with opposite spins.
- Hund’s Rule: When multiple molecular orbitals have the same energy, electrons will occupy them singly with parallel spins before pairing up.
These facets of Molecular Orbital Theory provide a comprehensive understanding of the electronic structure of CO2, enabling us to calculate its bond order accurately and gain insights into its chemical properties.
Valence Electrons
Valence electrons play a critical role in determining the bond order of a molecule, including CO2. Valence electrons are the electrons in the outermost shell of an atom, and they participate in chemical bonding. The number of valence electrons determines the number of bonds an atom can form.
In the case of CO2, each carbon atom has four valence electrons, and each oxygen atom has six valence electrons. To calculate the bond order, we need to consider the total number of valence electrons involved in the bonding between the atoms. In CO2, there are a total of 16 valence electrons: four from each carbon atom and six from each oxygen atom.
These valence electrons are used to form two double bonds between the carbon atom and each oxygen atom. A double bond consists of two pairs of electrons shared between two atoms, so each double bond contributes two to the bond order. Therefore, the bond order of CO2 is two.
Understanding the relationship between valence electrons and bond order is essential for predicting the structure and properties of molecules. By considering the number and arrangement of valence electrons, we can gain insights into the chemical bonding and behavior of various substances.
Hybridization
Hybridization is a fundamental concept in chemistry that describes the mixing of atomic orbitals to form new hybrid orbitals with different shapes and energies. In the context of calculating bond order for CO2, hybridization plays a crucial role in determining the geometry and properties of the molecule.
- sp Hybridization: In CO2, the carbon atom undergoes sp hybridization, mixing one 2s and one 2p orbital to form two equivalent sp hybrid orbitals. These hybrid orbitals are oriented in a linear fashion, with an angle of 180 between them.
- p Orbitals: The remaining two 2p orbitals on the carbon atom do not participate in hybridization and are perpendicular to the sp hybrid orbitals.
- Double Bonds: The sp hybrid orbitals from the carbon atom overlap with the p orbitals from the oxygen atoms, forming two double bonds. Each double bond consists of one sigma bond and one pi bond.
- Molecular Geometry: The linear arrangement of the sp hybrid orbitals and the presence of the double bonds result in the linear molecular geometry of CO2.
Understanding hybridization allows us to rationalize the bonding and molecular properties of CO2. By considering the hybridization of the carbon atom, we can determine the geometry of the molecule, the number of sigma and pi bonds, and the overall electronic structure, which are essential factors for calculating bond order accurately.
Resonance
In the context of bond order calculations for CO2, resonance plays a crucial role in describing the delocalization of electrons within the molecule. Resonance theory proposes that certain molecules cannot be accurately represented by a single Lewis structure but rather by a weighted average of multiple contributing structures.
- Equivalent Resonance Structures: CO2 exhibits resonance between two equivalent Lewis structures, each depicting a double bond between the carbon atom and one of the oxygen atoms. These structures contribute equally to the overall resonance hybrid.
- Delocalized Electrons: The double bonds in the resonance structures can be thought of as delocalized, meaning that the electrons are not confined to a specific bond but are spread out over the entire molecule. This delocalization results in a more stable and lower-energy molecular structure.
- Average Bond Order: The bond order in CO2, calculated as the average of the bond orders in the contributing resonance structures, is 1.5. This value indicates the partial double bond character of the carbon-oxygen bonds, reflecting the delocalization of electrons.
- Implications for Properties: Resonance in CO2 influences various molecular properties, such as bond lengths, vibrational frequencies, and reactivity. The delocalized electrons enhance the stability of the molecule and affect its interactions with other species.
By considering resonance, we gain a more accurate understanding of the electronic structure and properties of CO2. The delocalization of electrons through resonance contributes to the stability and unique behavior of this important molecule.
Bond Length
In the context of calculating bond order for CO2, bond length plays a crucial role in determining the strength and nature of the chemical bond between the carbon and oxygen atoms.
Bond length is inversely related to bond order. A shorter bond length typically indicates a stronger bond and a higher bond order. In CO2, the bond length between the carbon and oxygen atoms is approximately 1.16 angstroms, which corresponds to a bond order of 2.
Understanding the relationship between bond length and bond order is essential for predicting and explaining various chemical properties and behaviors. For instance, shorter bond lengths generally lead to higher bond strengths, influencing molecular stability, reactivity, and spectroscopic properties.
In summary, bond length is a critical component of calculating bond order for CO2, providing valuable insights into the electronic structure, bonding characteristics, and overall properties of the molecule.
Bond Strength
Bond strength, a measure of the force required to break a chemical bond, is a fundamental property that influences the stability, reactivity, and properties of molecules. In the context of calculating bond order for CO2, bond strength plays a crucial role in determining the nature of the chemical bond between the carbon and oxygen atoms.
The bond strength in CO2 is directly related to its bond order. A higher bond order indicates a stronger bond and, consequently, a higher bond strength. In CO2, the bond order is 2, which corresponds to a double bond between the carbon and each oxygen atom. This double bond results in a strong bond with a relatively short bond length and high bond strength.
Understanding the relationship between bond strength and bond order is essential for predicting and explaining various chemical phenomena. For example, molecules with stronger bonds are generally more stable and less reactive. They also exhibit distinct spectroscopic properties and can influence the physical properties of materials.
In summary, bond strength is a critical component of calculating bond order for CO2, providing insights into the strength and nature of the chemical bond. This understanding has practical applications in diverse fields, including chemistry, materials science, and biochemistry, guiding the design and development of new materials and technologies.
Magnetic Properties
Magnetic properties are not directly related to calculating bond order for CO2, as CO2 is a non-magnetic molecule. Bond order calculations primarily consider the electronic structure and bonding interactions between atoms, focusing on the number and type of shared electron pairs. Magnetic properties, on the other hand, arise from the presence of unpaired electrons and their interactions within a molecule or material. Therefore, magnetic properties are not a critical component of bond order calculations for CO2.
However, understanding magnetic properties is essential in other areas of chemistry, particularly for molecules and materials with unpaired electrons. Magnetic properties can provide insights into the electronic configuration, spin states, and interactions between paramagnetic species. This understanding has practical applications in various fields, such as magnetism, spintronics, and magnetic resonance imaging (MRI).
In summary, while magnetic properties are not directly involved in calculating bond order for CO2, they are crucial for understanding the behavior of magnetic materials and have significant applications in different scientific and technological domains.
Oxidation States
In the context of calculating bond order for CO2, understanding oxidation states provides valuable insights into the distribution of electrons and the nature of chemical bonding. Oxidation states describe the hypothetical charge of an atom in a molecule, assuming that all bonds are ionic.
- Assignment of Oxidation States: Oxidation states can be assigned to each atom in CO2 based on the electronegativity difference between the atoms and the number of electrons involved in bonding.
- Carbon’s Oxidation State: In CO2, the carbon atom has an oxidation state of +4, indicating that it has lost four electrons. This is consistent with the four single bonds that carbon forms with the two oxygen atoms.
- Oxygen’s Oxidation State: Each oxygen atom in CO2 has an oxidation state of -2, indicating that it has gained two electrons. This corresponds to the two double bonds that each oxygen atom forms with the carbon atom.
- Relation to Bond Order: The oxidation states of carbon and oxygen in CO2 are consistent with the bond order of 2, which indicates the presence of two double bonds. This demonstrates the relationship between oxidation states and bond order in covalent molecules.
By considering oxidation states, we gain a deeper understanding of the electron distribution and bonding interactions within CO2. This knowledge is essential for accurately calculating bond order and predicting the chemical properties and reactivity of the molecule.
Molecular Geometry
Molecular geometry plays a crucial role in how to calculate bond order for CO2. The geometry of a molecule, which describes the spatial arrangement of its atoms, is determined by the number and type of bonds between the atoms. In the case of CO2, the molecular geometry is linear, with the carbon atom bonded to two oxygen atoms in a straight line.
The linear geometry of CO2 is a direct consequence of its bond order. The bond order between the carbon atom and each oxygen atom is 2, indicating the presence of two double bonds. These double bonds result in a strong repulsion between the electron pairs in the bonding orbitals, forcing the molecule to adopt a linear geometry to minimize the repulsion.
Understanding the relationship between molecular geometry and bond order is essential for accurately calculating bond order for CO2 and other molecules. By considering the geometry of the molecule, we can infer the number and type of bonds between the atoms, which in turn allows us to calculate the bond order.
Spectroscopic Properties
Spectroscopic properties refer to the interactions between molecules and electromagnetic radiation. They provide valuable insights into the electronic structure, bonding, and molecular dynamics of CO2, which are crucial for calculating bond order accurately.
- Vibrational Spectroscopy:
When CO2 molecules absorb infrared radiation, the bonds between the atoms vibrate. By analyzing the vibrational frequencies and intensities, we can deduce the bond strengths and force constants, which are related to bond order. - Electronic Spectroscopy:
Ultraviolet and visible radiation can excite electrons in CO2 molecules, leading to electronic transitions. The energy difference between these transitions corresponds to the energy levels of the molecular orbitals, providing insights into the bond order and electronic structure. - Raman Spectroscopy:
Raman spectroscopy involves inelastic scattering of light by molecules. The vibrational and rotational energy levels of CO2 can be probed using Raman spectroscopy, providing information about bond lengths and angles, which are related to bond order. - NMR Spectroscopy:
Nuclear magnetic resonance (NMR) spectroscopy can be used to study the local electronic environment around specific atoms in CO2. By analyzing the chemical shifts and coupling constants, we can infer information about bond order and hybridization, which are interconnected concepts.
In summary, spectroscopic properties offer a multifaceted approach to understanding the electronic structure and bonding in CO2. By combining various spectroscopic techniques, we can obtain a comprehensive picture of the molecule, including its bond order, which is a fundamental parameter for predicting its chemical behavior and reactivity.
FAQs on Calculating Bond Order for CO2
This section addresses frequently asked questions and clarifies essential concepts related to calculating bond order for CO2 to enhance understanding.
Question 1: What is bond order and why is it significant?
Answer: Bond order describes the strength and nature of the chemical bond between atoms. It is a crucial parameter for understanding molecular stability, reactivity, and various properties.
Question 2: How do I determine the molecular orbital configuration for CO2?
Answer: The molecular orbital configuration can be determined using molecular orbital theory, considering the linear combination of atomic orbitals, Aufbau principle, Pauli exclusion principle, and Hund’s rule.
Question 3: How does hybridization affect bond order in CO2?
Answer: Hybridization involves the mixing of atomic orbitals to form new hybrid orbitals. In CO2, sp hybridization of the carbon atom results in a linear molecular geometry and influences the bond order.
Question 4: What is the role of resonance in bond order calculations for CO2?
Answer: Resonance theory proposes that CO2 exists as a hybrid of multiple contributing structures, leading to the delocalization of electrons and an average bond order of 1.5.
Question 5: How is bond length related to bond order in CO2?
Answer: Bond length is inversely related to bond order. The shorter bond length in CO2, approximately 1.16 angstroms, corresponds to a bond order of 2, indicating a strong double bond.
Question 6: What are the implications of bond order for CO2’s chemical properties?
Answer: Bond order influences various chemical properties of CO2, including bond strength, molecular geometry, spectroscopic properties, and reactivity, providing insights into its behavior and applications.
These FAQs provide fundamental insights into calculating bond order for CO2. Understanding these concepts is essential for accurately predicting and rationalizing the properties and behavior of CO2 in diverse chemical systems.
In the following sections, we will delve deeper into applications of bond order calculations, exploring how it aids in comprehending molecular structure, reactivity, and spectroscopy.
Tips for Calculating Bond Order for CO2
To enhance your understanding and accuracy in calculating bond order for CO2, consider these practical tips:
Tip 1: Begin by comprehending the fundamental concepts of bond order, including its definition and significance in describing the strength and nature of chemical bonds.
Tip 2: Familiarize yourself with molecular orbital theory, which provides a theoretical framework for understanding the electronic structure of molecules and the formation of molecular orbitals.
Tip 3: Determine the hybridization of the carbon atom in CO2, as it influences the molecular geometry and the number of sigma and pi bonds formed.
Tip 4: Consider resonance theory to account for the delocalization of electrons in CO2, which affects the bond order and molecular properties.
Tip 5: Utilize experimental data, such as bond lengths and spectroscopic information, to validate your calculated bond order and gain insights into the molecular structure.
Tip 6: Understand the relationship between bond order and bond strength, as a higher bond order generally corresponds to a stronger bond and greater stability.
Tip 7: Explore the impact of bond order on molecular geometry, as it influences the spatial arrangement of atoms and the overall shape of the molecule.
Tip 8: Apply bond order calculations to predict and explain the chemical reactivity and properties of CO2, including its role in various chemical processes and applications.
These tips serve as valuable guidelines for accurately calculating bond order for CO2, enabling you to gain a deeper understanding of its molecular structure, bonding characteristics, and chemical behavior.
In the concluding section, we will explore advanced applications of bond order calculations, demonstrating their significance in diverse areas of chemistry and beyond.
Conclusion
Throughout this article, we have explored the intricate world of bond order calculations for carbon dioxide (CO2). By delving into the concepts of molecular orbital theory, hybridization, resonance, and various experimental techniques, we have gained a comprehensive understanding of the factors that influence bond order in CO2.
Key insights from this exploration include the recognition that bond order is directly related to bond strength and molecular geometry. Additionally, resonance plays a crucial role in CO2, as it contributes to the delocalization of electrons and affects the overall bond order. These concepts are interconnected and provide a holistic view of the electronic structure and chemical behavior of CO2.
The ability to accurately calculate bond order for CO2 is not only essential for comprehending its molecular properties but also opens avenues for further research and applications. By understanding bond order, scientists can delve deeper into the reactivity, spectroscopy, and diverse chemical processes involving CO2, leading to advancements in fields such as atmospheric chemistry, materials science, and energy conversion.
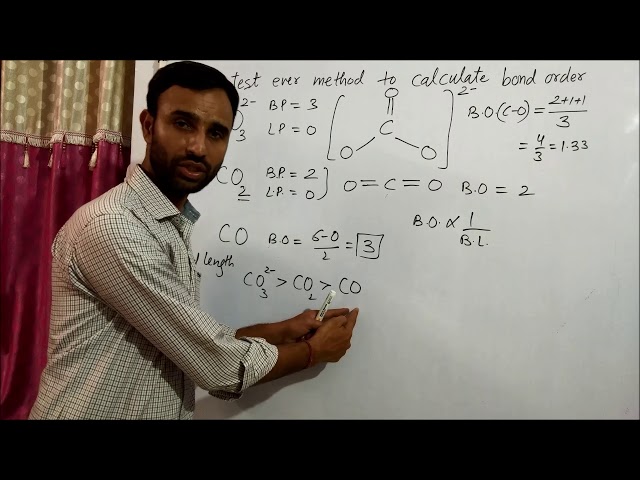