How to Calculate Bond Order of CO refers to a chemical procedure used to determine the strength and stability of the bond between carbon and oxygen atoms in a carbon monoxide (CO) molecule. It involves applying specific equations and considering various factors, such as the number of electrons involved in the bond and the molecular orbital interactions.
Understanding bond order is essential in chemistry as it provides insights into the properties and reactivity of molecules. For instance, a higher bond order generally indicates a stronger and more stable bond, influencing factors such as molecular geometry, bond length, and vibrational frequency. The concept of bond order was first introduced by Linus Pauling in 1931 and has since become a fundamental tool in understanding chemical bonding.
This article will delve into the detailed steps and formulas involved in calculating the bond order of CO, exploring its significance in understanding the nature of chemical bonds and molecular behavior.
How to Calculate Bond Order of CO
Understanding the essential aspects of calculating bond order in carbon monoxide (CO) is crucial for comprehending the nature of chemical bonding and molecular behavior. These key aspects encompass various dimensions, including:
- Molecular orbital theory
- Electron configuration
- Bond length
- Bond strength
- Molecular geometry
- Quantum mechanics
- Resonance
- Hybridization
- Delocalization
- Valence bond theory
These aspects are interconnected and provide a comprehensive framework for calculating bond order in CO. By considering factors such as the number of electrons involved in bonding, the overlap of atomic orbitals, and the molecular symmetry, chemists can accurately determine the strength and stability of the CO bond. This knowledge is essential for understanding various chemical phenomena, including molecular reactivity, spectroscopic properties, and thermodynamic behavior.
Molecular Orbital Theory
Molecular orbital theory plays a pivotal role in understanding the electronic structure of molecules and calculating bond order, including that of carbon monoxide (CO). It provides a conceptual framework for describing the distribution and behavior of electrons within a molecule, offering valuable insights into chemical bonding and molecular properties.
- Atomic Orbitals: The foundation of molecular orbital theory lies in atomic orbitals, which describe the wave-like behavior of electrons around individual atoms. These orbitals are characterized by their shape, energy, and the number of electrons they can hold.
- Linear Combination of Atomic Orbitals (LCAO): Molecular orbitals are formed by combining atomic orbitals from the constituent atoms. The LCAO approach involves adding and subtracting atomic orbitals to create new orbitals that extend over the entire molecule.
- Molecular Orbital Energy Levels: The energy levels of molecular orbitals are determined by the energies of the atomic orbitals from which they are formed. The lowest energy molecular orbitals are filled first, following the Aufbau principle.
- Bonding and Antibonding Orbitals: Molecular orbitals can be classified as bonding or antibonding based on their effect on the overlap of atomic orbitals. Bonding orbitals result from constructive interference, leading to increased electron density between the nuclei, while antibonding orbitals arise from destructive interference, reducing electron density.
By considering the molecular orbital interactions and the number of electrons occupying bonding and antibonding orbitals, chemists can calculate the bond order of CO. This information provides insights into the strength and stability of the CO bond, helping to understand its chemical reactivity and spectroscopic properties.
Electron Configuration
Electron configuration is a crucial aspect of calculating bond order in carbon monoxide (CO) as it determines the number and arrangement of electrons involved in the chemical bond. Each atom contributes its valence electrons to form molecular orbitals, and the distribution of these electrons affects the overall bond strength and stability.
In CO, the carbon atom has six valence electrons (2s22p2), while the oxygen atom has four valence electrons (2s22p4). When these atoms combine to form CO, their valence electrons interact to form molecular orbitals. The molecular orbital diagram for CO shows that the bonding molecular orbital (2p) is formed by the overlap of the 2p orbitals from carbon and oxygen. This orbital is filled with two electrons, one from each atom.
The bond order of CO can be calculated using the formula: Bond order = (number of electrons in bonding orbitals – number of electrons in antibonding orbitals) / 2 In the case of CO, there are two electrons in the bonding orbital and zero electrons in the antibonding orbital, resulting in a bond order of 1. This indicates a single bond between carbon and oxygen.
Understanding the electron configuration of CO is essential for accurately calculating its bond order, which provides insights into the molecule’s chemical properties and reactivity. This understanding finds applications in various fields, including catalysis, spectroscopy, and atmospheric chemistry.
Bond Length
Bond length is a fundamental aspect of “how to calculate bond order of CO” as it provides insights into the strength and stability of the bond between carbon and oxygen atoms. A shorter bond length generally indicates a stronger bond, while a longer bond length suggests a weaker bond.
- Atomic Radii: The atomic radii of carbon and oxygen influence the bond length in CO. Carbon has a smaller atomic radius than oxygen, resulting in a shorter bond length.
- Bond Order: Bond order is inversely related to bond length. A higher bond order indicates a shorter bond length due to increased electron density between the atoms.
- Hybridization: The hybridization of the carbon atom in CO affects the bond length. sp-Hybridized carbon forms a shorter bond than sp2– or sp3-hybridized carbon.
- Resonance: Resonance, which involves the delocalization of electrons, can affect bond length. Delocalized electrons can strengthen bonds, leading to shorter bond lengths.
By considering these factors that influence bond length, chemists can gain valuable insights into the nature of the CO bond and its properties. Understanding bond length is crucial for predicting molecular behavior, reactivity, and spectroscopic characteristics.
Bond Strength
Bond strength is a critical aspect of “how to calculate bond order of CO” as it quantifies the strength of the bond between carbon and oxygen atoms, providing insights into the molecule’s stability and reactivity. Several factors influence bond strength, including:
- Bond Order: Bond order is directly proportional to bond strength. A higher bond order indicates a stronger bond due to increased electron density between the atoms.
- Electronegativity: The difference in electronegativity between carbon and oxygen contributes to bond strength. The greater the electronegativity difference, the stronger the bond due to the attraction between opposite charges.
- Bond Length: Bond strength is inversely related to bond length. A shorter bond length generally indicates a stronger bond due to increased overlap between atomic orbitals.
- Resonance: Resonance, which involves the delocalization of electrons, can affect bond strength. Delocalized electrons can strengthen bonds by distributing the electron density over multiple atoms.
Understanding bond strength is crucial for predicting molecular behavior and reactivity. A stronger bond generally leads to a more stable molecule, less likely to undergo chemical reactions. Conversely, a weaker bond suggests a more reactive molecule, more prone to breaking and forming new bonds. By considering the factors that influence bond strength, chemists can gain valuable insights into the nature of the CO bond and its properties.
Molecular geometry
Molecular geometry is a key aspect of “how to calculate bond order of CO” as it provides insights into the three-dimensional arrangement of atoms within the molecule. This arrangement influences various properties of the molecule, including its bond strength, reactivity, and spectroscopic characteristics.
- Linear Structure: CO has a linear molecular geometry, with the carbon and oxygen atoms bonded together by a single bond. This linear structure is a consequence of the sp-hybridization of the carbon atom.
- Bond Angle: The bond angle in CO is 180 degrees, indicating that the molecule is linear. This bond angle is a result of the sp-hybridized carbon atom and the lone pairs of electrons on the oxygen atom.
- Dipole Moment: CO has a polar covalent bond due to the difference in electronegativity between carbon and oxygen. This polarity results in a dipole moment, with the positive end of the dipole on the carbon atom and the negative end on the oxygen atom.
- Molecular Orbitals: The molecular orbitals of CO can be described using molecular orbital theory. The bonding molecular orbital (2p) is formed by the overlap of the 2p orbitals from carbon and oxygen. This orbital is filled with two electrons, one from each atom.
Understanding molecular geometry is essential for accurately calculating the bond order of CO and predicting its properties. The linear structure, bond angle, dipole moment, and molecular orbitals all contribute to the unique characteristics of CO and its behavior in chemical reactions.
Quantum mechanics
Quantum mechanics plays a pivotal role in understanding the electronic structure of molecules and calculating their properties, including bond order. It provides a theoretical framework for describing the behavior of electrons and their interactions within atoms and molecules.
In the context of “how to calculate bond order of CO,” quantum mechanics is essential for determining the number and types of molecular orbitals formed by the overlap of atomic orbitals. These molecular orbitals can be bonding, antibonding, or non-bonding, and their energies and occupancies determine the stability and properties of the molecule. By applying quantum mechanical principles, chemists can calculate the bond order of CO, which is a measure of the strength and stability of the bond between carbon and oxygen atoms.
A practical application of quantum mechanics in calculating bond order is in the field of computational chemistry. Computational methods, such as density functional theory (DFT) and Hartree-Fock theory, utilize quantum mechanical principles to calculate the electronic structure of molecules and determine their properties, including bond order. These methods are widely used in various scientific disciplines, including chemistry, physics, and materials science, to study and predict the behavior of molecules and materials.
In summary, quantum mechanics is a fundamental component of “how to calculate bond order of CO” as it provides the theoretical basis for understanding the electronic structure and bonding in molecules. By applying quantum mechanical principles, chemists can accurately determine bond order, providing insights into the strength, stability, and properties of chemical bonds.
Resonance
Resonance is a crucial concept in chemistry that plays a significant role in understanding “how to calculate bond order of CO.” It refers to the phenomenon where multiple Lewis structures can be drawn for a molecule, each representing a different distribution of electrons. These resonance structures contribute to the overall electronic structure and properties of the molecule.
In the case of CO, resonance is particularly important because it affects the bond order. Carbon monoxide can be represented by two resonance structures: one with a double bond between carbon and oxygen, and the other with a triple bond. The actual electronic structure of CO is a hybrid of these two resonance structures, resulting in a bond order of 2.5. This means that the bond between carbon and oxygen in CO is stronger than a single bond but weaker than a double bond.
Understanding resonance is essential for accurately calculating the bond order of CO. By considering the resonance structures and their contributions to the overall electronic structure, chemists can determine the most accurate bond order, which provides valuable insights into the strength and stability of the CO bond. This understanding finds applications in various fields, including catalysis, spectroscopy, and atmospheric chemistry.
In summary, resonance is a critical component of “how to calculate bond order of CO” as it allows chemists to consider the different electronic structures that contribute to the overall bonding in the molecule. By understanding resonance, chemists can accurately determine bond order, which provides valuable insights into the properties and behavior of molecules.
Hybridization
In the context of “how to calculate bond order of CO,” hybridization is a fundamental concept that describes the mixing of atomic orbitals to form new hybrid orbitals with different shapes and energies. This hybridization plays a crucial role in determining the geometry, bonding, and overall properties of the CO molecule.
- sp Hybridization: In CO, the carbon atom undergoes sp hybridization, where one 2s orbital and one 2p orbital combine to form two sp hybrid orbitals. These hybrid orbitals are oriented 180 apart, resulting in the linear geometry of CO.
- Overlap and Bonding: The sp hybrid orbital on carbon overlaps with the 2p orbital on oxygen, forming a sigma bond. This sigma bond is the primary contributor to the bond order of CO.
- Triple Bond Character: Due to the sp hybridization of carbon, CO exhibits triple bond character. The two sp hybrid orbitals on carbon can form two sigma bonds and two pi bonds with the oxygen atom, resulting in a bond order of 3.
- Stability and Reactivity: The sp hybridization of carbon in CO contributes to the molecule’s stability and low reactivity. The linear geometry minimizes steric hindrance, and the triple bond character makes CO less susceptible to bond breaking.
Understanding hybridization is essential for accurately calculating the bond order of CO. By considering the hybridization of the carbon atom and the overlap of atomic orbitals, chemists can determine the number and types of bonds formed, providing insights into the molecular structure and properties of CO.
Delocalization
In the context of “how to calculate bond order of CO,” delocalization plays a crucial role in understanding the electronic structure and properties of the molecule. Delocalization refers to the spreading out or distribution of electrons over multiple atoms or molecular orbitals, rather than being confined to a specific bond or atom.
In CO, delocalization occurs due to the resonance between the two resonance structures: one with a double bond between carbon and oxygen, and the other with a triple bond. This resonance leads to the delocalization of the electrons over both the carbon and oxygen atoms, resulting in a bond order of 2.5, which is intermediate between a double and triple bond.
Understanding delocalization is critical for accurately calculating the bond order of CO. By considering the delocalization of the electrons, chemists can determine the most accurate bond order, which provides valuable insights into the strength and stability of the CO bond. This understanding finds applications in various fields, including catalysis, spectroscopy, and atmospheric chemistry.
In summary, delocalization is a key component of “how to calculate bond order of CO” as it allows chemists to consider the resonance structures and the delocalization of electrons. By understanding delocalization, chemists can accurately determine bond order, which provides valuable insights into the properties and behavior of molecules.
Valence bond theory
Valence bond theory is a fundamental concept in chemistry that provides a framework for understanding and calculating the bond order of molecules, including carbon monoxide (CO). It focuses on the interactions and hybridization of atomic orbitals to form chemical bonds.
- Atomic Orbitals: Valence bond theory describes the formation of bonds based on the overlap of atomic orbitals. In CO, the carbon atom contributes its 2s and 2p orbitals, while the oxygen atom contributes its 2s and 2p orbitals.
- Hybridization: The carbon atom in CO undergoes sp hybridization, mixing its 2s and 2p orbitals to form two sp hybrid orbitals. These hybrid orbitals overlap with the 2p orbitals of oxygen to form the sigma bond.
- Bond Order: The bond order in CO is determined by counting the number of electron pairs shared between the atoms. In CO, the sigma bond involves two shared electrons, resulting in a bond order of 1.
- Resonance: Valence bond theory also considers resonance, which involves the delocalization of electrons between different Lewis structures. In CO, resonance contributes to the stability of the molecule and affects its bond order.
By considering these aspects of valence bond theory, chemists can gain valuable insights into the electronic structure and bonding of CO. This understanding enables accurate calculation of bond order and provides a foundation for studying various chemical phenomena involving CO.
Frequently Asked Questions
This section addresses common questions and clarifies key concepts related to calculating the bond order of carbon monoxide (CO).
Question 1:What is the significance of bond order in understanding CO?
Answer: Bond order provides insights into the strength, stability, and electronic structure of the CO molecule. It helps predict molecular properties such as bond length, vibrational frequency, and reactivity.
Question 2:How is the bond order of CO calculated?
Answer: The bond order of CO is calculated using the formula: Bond order = (Number of electrons in bonding orbitals – Number of electrons in antibonding orbitals) / 2.
Question 3:What factors influence the bond order of CO?
Answer: Factors affecting bond order include the number of electrons involved in bonding, the hybridization of atomic orbitals, and the presence of resonance.
Question 4:What is the relationship between bond order and bond strength?
Answer: Bond order is directly proportional to bond strength. A higher bond order indicates a stronger bond due to increased electron density and overlap between atomic orbitals.
Question 5:How does resonance affect the bond order of CO?
Answer: Resonance delocalizes electrons over multiple bonds, resulting in a fractional bond order. In CO, resonance contributes to the stability of the molecule and increases its bond order.
Question 6:Why is it important to consider molecular orbital theory when calculating bond order?
Answer: Molecular orbital theory provides a framework for understanding the electronic structure of molecules. It helps identify the bonding and antibonding orbitals involved in CO, which is crucial for calculating the bond order accurately.
These FAQs provide essential insights into the calculation and significance of bond order in CO, laying the foundation for further exploration of its chemical properties and behavior.
Transition to the next section: In the following section, we will delve deeper into the applications of bond order in understanding CO’s reactivity, spectroscopic characteristics, and its role in various chemical processes.
Tips for Calculating Bond Order of CO
This section provides practical tips to enhance your understanding and accuracy when calculating the bond order of carbon monoxide (CO).
Tip 1: Identify the atomic orbitals involved in bonding. For CO, the carbon atom contributes its sp hybrid orbitals, while oxygen contributes its 2p orbitals.
Tip 2: Determine the number of electrons involved in bonding and antibonding orbitals. CO has four bonding electrons and zero antibonding electrons.
Tip 3: Use the formula: Bond order = (Number of electrons in bonding orbitals – Number of electrons in antibonding orbitals) / 2.
Tip 4: Consider resonance structures to determine the delocalization of electrons and its effect on bond order.
Tip 5: Understand the relationship between bond order and bond strength. A higher bond order indicates a stronger bond.
Tip 6: Utilize molecular orbital theory to visualize the bonding and antibonding orbitals and their contributions to bond order.
Tip 7: Practice calculating bond order for various CO-containing molecules to enhance your proficiency.
Tip 8: Consult reliable textbooks or online resources for further guidance and examples.
Following these tips will empower you to confidently calculate bond order for CO, providing valuable insights into its chemical properties and behavior.
In the concluding section, we will explore practical applications of bond order in understanding CO’s reactivity, spectroscopy, and its significance in various chemical processes.
Conclusion
This comprehensive guide on “how to calculate bond order of CO” has provided a detailed exploration of the theoretical foundations, practical applications, and significant implications of bond order in understanding CO’s chemical behavior. Key insights gained include the influence of molecular orbital interactions, electron configurations, and resonance on bond order determination.
The strong correlation between bond order and bond strength highlights its importance in predicting molecular stability and reactivity. Furthermore, the understanding of bond order enables chemists to tailor CO’s properties for specific applications, such as catalysis, spectroscopy, and atmospheric chemistry.
As we continue to unravel the intricacies of chemical bonding, the concept of bond order remains a cornerstone in comprehending and manipulating molecular systems. Its versatility and predictive power empower scientists to design new materials, optimize chemical reactions, and address global challenges related to energy, sustainability, and human health.
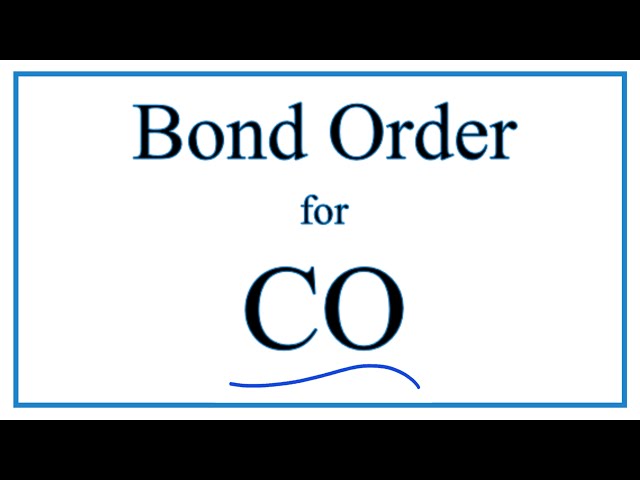