Bond order, a crucial concept in chemistry, describes the number of chemical bonds between two atoms. Understanding how to calculate the bond order of CN– (cyanide ion) is essential for comprehending its chemical behavior and reactivity. In various fields, including materials science and pharmaceutical research, bond order plays a vital role in designing and developing new materials and drugs.
Bond order determination has advanced our knowledge of chemical bonding and molecular structure. Historically, Linus Pauling’s pioneering work on the valence bond theory laid the foundation for understanding bond order, enabling chemists to predict and explain the properties of molecules.
This article delves into the intricacies of calculating the bond order of CN–, exploring the underlying principles, methods, and applications. By gaining a comprehensive understanding of bond order calculations, readers will be equipped to tackle complex chemical problems and make informed decisions in their research endeavors.
How to Calculate Bond Order of CN–
Calculating the bond order of CN– (cyanide ion) plays a crucial role in understanding its chemical bonding and reactivity. Key aspects to consider include:
- Atomic orbitals involved
- Molecular symmetry
- Electron configuration
- Hybridization
- Resonance
- Bond length
- Bond strength
- Molecular orbital theory
- Valence bond theory
- Quantum mechanics
These aspects are interconnected and provide valuable insights into the nature of the CN– bond. By understanding these concepts, chemists can predict and explain the chemical behavior of cyanide ion and its derivatives, which have applications in various fields such as coordination chemistry, organometallic chemistry, and biochemistry.
Atomic orbitals involved
In the context of calculating the bond order of CN– (cyanide ion), understanding the atomic orbitals involved is paramount. These orbitals, which describe the electron distribution around atoms, play a crucial role in determining the nature and strength of the chemical bond.
- Atomic Orbital Overlap
The extent to which the atomic orbitals of the bonded atoms overlap determines the strength of the bond. Greater overlap leads to stronger bonds and higher bond order.
- Orbital Symmetry
The symmetry of the atomic orbitals involved affects the bonding interaction. Orbitals with matching symmetry can overlap more effectively, resulting in stronger bonds.
- Hybridization
Hybridization, the mixing of atomic orbitals to form new hybrid orbitals, can influence the overlap and symmetry of the orbitals involved in bonding, thereby affecting the bond order.
- Resonance
In molecules with resonance, multiple Lewis structures can be drawn, each representing a different arrangement of electrons in the atomic orbitals. Resonance can affect the bond order by delocalizing electrons, leading to fractional bond orders.
These factors collectively determine the atomic orbitals involved in the CN– bond and influence the overall bond order. Understanding these aspects provides a deeper insight into the electronic structure and bonding characteristics of cyanide ion.
Molecular symmetry
Molecular symmetry plays a pivotal role in comprehending the bond order of CN– (cyanide ion) and its chemical properties. Symmetry operations, such as rotations, reflections, and inversions, describe the symmetry of a molecule, and they have important implications for bond order calculations.
- Point Group Symmetry
Point group symmetry classifies molecules based on their symmetry operations. The point group of CN– is Cv, which indicates its cylindrical symmetry.
- Molecular Orbitals and Symmetry
Molecular orbitals, which describe the electron distribution in a molecule, must be compatible with the molecular symmetry. The symmetry of the orbitals involved in the CN– bond determines the type and strength of the bond.
- Bonding and Antibonding Orbitals
Symmetry can differentiate between bonding orbitals, which promote bonding, and antibonding orbitals, which weaken bonding. In CN–, the bonding and antibonding orbitals are and *, respectively.
- Resonance and Symmetry
Resonance, the delocalization of electrons across multiple Lewis structures, affects the symmetry of the molecule. In CN–, resonance contributes to the overall bond order and the stability of the ion.
These aspects of molecular symmetry collectively influence the bond order of CN–, enabling chemists to understand its electronic structure and bonding characteristics. By considering the symmetry of the molecule and its constituent orbitals, we can gain deeper insights into the nature of the CN– bond and its behavior in various chemical reactions.
Electron configuration
Electron configuration, the distribution of electrons in atomic orbitals, is a fundamental aspect in determining the bond order of CN– (cyanide ion). It provides insights into the electronic structure and bonding characteristics of the ion.
- Atomic Orbitals
Electron configuration describes the arrangement of electrons in atomic orbitals, which are specific regions around the nucleus where electrons are most likely to be found. The type and number of atomic orbitals involved in bonding determine the strength and properties of the bond.
- Valence Electrons
Valence electrons are the electrons in the outermost shell of an atom, which participate in chemical bonding. The number of valence electrons and their arrangement determine the bonding behavior and reactivity of the atom.
- Octet Rule
The octet rule states that atoms tend to gain or lose electrons to achieve a stable electron configuration with eight valence electrons. This rule influences the bond order of CN–, as the carbon atom in cyanide ion strives to satisfy the octet rule.
- Molecular Orbitals
Electron configuration also contributes to the formation of molecular orbitals, which describe the electron distribution in a molecule. The overlap and interaction of atomic orbitals result in the formation of molecular orbitals, which determine the bond order and properties of the molecule.
In summary, the electron configuration of the atoms involved in the CN– bond, particularly the valence electrons and their arrangement, plays a crucial role in determining the bond order. Understanding electron configuration provides valuable insights into the electronic structure, bonding behavior, and reactivity of cyanide ion.
Hybridization
Hybridization, a crucial aspect of understanding how to calculate the bond order of CN– (cyanide ion), involves the intermixing of atomic orbitals to form new hybrid orbitals with different shapes and energies. This process plays a significant role in determining the geometry, bonding, and properties of the molecule.
- Types of Hybridization
Various types of hybridization can occur, including sp, sp2, sp3, and sp3d, depending on the number and type of atomic orbitals involved.
- Geometry and Bonding
Hybridization influences the geometry of the molecule by determining the arrangement of the hybrid orbitals around the central atom. This, in turn, affects the bond angles, bond lengths, and overall shape of the molecule.
- Molecular Orbital Formation
Hybrid orbitals participate in the formation of molecular orbitals, which describe the electron distribution in the molecule. The type and symmetry of the hybrid orbitals involved determine the nature and strength of the chemical bonds formed.
In the context of CN–, hybridization helps explain the bonding and geometry of the ion. The carbon atom in CN– undergoes sp hybridization, resulting in the formation of two hybrid orbitals that overlap with the nitrogen atom’s p orbitals to form the CN bond. This hybridization contributes to the linear geometry and strong bond order of the cyanide ion.
Resonance
Resonance, a fundamental concept in chemistry, plays a crucial role in understanding how to calculate the bond order of CN– (cyanide ion). It depicts the delocalization of electrons across multiple Lewis structures, providing valuable insights into the bonding and properties of the ion.
- Equivalent Lewis Structures
Resonance involves drawing multiple Lewis structures for a molecule or ion, each representing a different arrangement of electrons. These structures are equivalent in terms of energy and contribute to the resonance hybrid. - Delocalized Electrons
In resonance structures, electrons are delocalized, meaning they are not confined to a specific atom or bond. This delocalization results in a more stable and lower energy state for the molecule. - Bond Order and Resonance
Resonance affects the bond order of CN– by delocalizing the electrons involved in the bond. This delocalization reduces the bond order compared to a single Lewis structure, making the bond less localized and stronger. - Implications for Reactivity
The delocalized electrons in resonance structures influence the reactivity of CN–. The ion’s stability and reduced bond order make it less reactive than expected based on a single Lewis structure.
In summary, resonance is a crucial aspect in understanding the bond order of CN–. It involves delocalized electrons and equivalent Lewis structures, leading to a more stable and less localized bond. This concept provides valuable insights into the bonding and properties of cyanide ion, enabling a deeper comprehension of its chemical behavior.
Bond Length
Bond length, the distance between the nuclei of two bonded atoms, plays a significant role in understanding and calculating the bond order of CN– (cyanide ion). It provides insights into the strength and nature of the chemical bond.
Bond length is inversely related to bond order. A shorter bond length indicates a higher bond order, which implies a stronger bond. This relationship arises from the fact that shorter bonds have a greater overlap between the electron clouds of the bonded atoms, leading to increased electron density and stronger bonding interactions.
In the case of CN–, the bond length between carbon and nitrogen is relatively short, indicating a high bond order. This strong bond is attributed to the triple bond character between carbon and nitrogen, resulting from the overlap of three pairs of electrons in three molecular orbitals.
Understanding the connection between bond length and bond order is crucial for predicting and explaining the chemical behavior of molecules. By considering bond lengths, chemists can gain insights into the strength, stability, and reactivity of chemical bonds, guiding the design and development of new materials and drugs.
Bond Strength
Within the context of calculating the bond order of CN–, bond strength plays a critical role in determining the stability and properties of the molecule. Bond strength, a measure of the force required to break a bond, is influenced by various factors, providing insights into the nature of the chemical bond.
- Bond Length
Bond strength is inversely proportional to bond length. Shorter bonds, with smaller bond lengths, indicate stronger bonds due to increased overlap between electron clouds.
- Bond Order
Bond strength is directly proportional to bond order. Higher bond orders, representing multiple bonds, result in stronger bonds due to increased electron density.
- Electronegativity
Electronegativity, the ability of an atom to attract electrons, influences bond strength. Bonds between atoms with similar electronegativity are stronger than those between atoms with significant electronegativity differences.
- Hybridization
Hybridization of atomic orbitals affects bond strength. Hybrid orbitals with greater overlap, such as sp3 hybridized orbitals, lead to stronger bonds.
Understanding bond strength is essential for predicting chemical reactivity and designing new materials. By considering these factors, chemists can tailor molecules with specific properties, optimize reaction conditions, and develop innovative applications based on bond strength.
Molecular orbital theory
In the realm of understanding how to calculate the bond order of CN– (cyanide ion), molecular orbital theory (MOT) stands as a fundamental pillar, providing a comprehensive framework for comprehending the electronic structure and bonding behavior of molecules.
- Linear Combination of Atomic Orbitals (LCAO)
MOT posits that molecular orbitals, which describe the distribution of electrons in a molecule, are formed by the linear combination of atomic orbitals. In the case of CN–, the 2pz orbital of carbon and the 2pz orbital of nitrogen combine to form the molecular orbitals.
- Molecular Orbital Energy Levels
MOT predicts that molecular orbitals have quantized energy levels, and the energy of each molecular orbital depends on the type and symmetry of the atomic orbitals that combine to form it.
- Bonding and Antibonding Orbitals
MOT distinguishes between bonding and antibonding molecular orbitals. Bonding orbitals promote electron density between the nuclei, leading to bond formation, while antibonding orbitals have electron density concentrated outside the internuclear region, weakening the bond.
- Population Analysis
MOT provides methods for calculating the electron population of molecular orbitals, which is crucial for determining the bond order. In the case of CN–, the electron population of the bonding orbital indicates the bond order of the CN bond.
By employing molecular orbital theory, chemists can gain deep insights into the electronic structure of CN–, rationalize its bonding behavior, and accurately calculate its bond order. MOT serves as a powerful tool for understanding and predicting the properties and reactivity of a wide range of chemical compounds.
Valence bond theory
In the context of calculating the bond order of CN– (cyanide ion), valence bond theory (VBT) offers a valuable framework for understanding the nature of the chemical bond. VBT focuses on the hybridization of atomic orbitals and the overlap of these orbitals to form molecular orbitals.
- Hybrid Orbitals
VBT explains how atomic orbitals can hybridize to form new hybrid orbitals with specific shapes and orientations. In CN–, the carbon atom undergoes sp hybridization, resulting in the formation of two hybrid orbitals that overlap with the nitrogen atom’s p orbitals to form the CN bond.
- Sigma and Pi Bonds
VBT distinguishes between sigma () bonds, which are formed by the head-to-head overlap of orbitals, and pi () bonds, which are formed by the lateral overlap of orbitals. In CN–, the CN bond is a bond.
- Bond Order
VBT provides a method for calculating the bond order of a molecule. Bond order is defined as the number of electron pairs shared between two atoms. In CN–, the bond order is 3, indicating a triple bond between carbon and nitrogen.
- Resonance
VBT can be used to explain resonance, which is the delocalization of electrons across multiple bonds. In CN–, resonance structures can be drawn to depict the delocalization of the electrons, which contributes to the stability of the ion.
By considering the hybridization of atomic orbitals, the types of bonds formed, and the concept of resonance, valence bond theory provides a comprehensive understanding of the electronic structure and bonding in CN–, enabling the accurate calculation of its bond order and providing insights into its chemical properties and reactivity.
Quantum mechanics
In the realm of calculating the bond order of CN– (cyanide ion), quantum mechanics emerges as a fundamental pillar, providing a theoretical framework that unveils the intricate details of atomic and molecular interactions.
- Wave-Particle Duality
Quantum mechanics posits that particles, such as electrons, exhibit both wave-like and particle-like properties. This duality influences the behavior of electrons in atoms and molecules, shaping the formation and properties of chemical bonds.
- Schrdinger’s Equation
The Schrdinger equation, a cornerstone of quantum mechanics, describes the wavefunction of a particle, providing information about its energy, momentum, and spatial distribution. Solving the Schrdinger equation for atoms and molecules allows chemists to determine the electronic structure and properties of these systems, including bond order.
- Quantum Superposition
Quantum superposition refers to the ability of a particle to exist in multiple states simultaneously. This concept plays a crucial role in understanding the bonding in CN–, as it allows for the delocalization of electrons across the molecule.
- Quantum Tunneling
Quantum tunneling describes the ability of particles to pass through potential energy barriers, even if their energy is lower than the barrier. This phenomenon can influence chemical reactions and bonding, including the formation of CN–.
By embracing the principles of quantum mechanics, chemists gain a deeper understanding of the electronic structure and bonding in CN–, enabling accurate bond order calculations and insights into the ion’s chemical properties and reactivity.
Frequently Asked Questions
This section addresses common questions and clarifies key aspects of calculating the bond order of CN–.
Question 1: What is bond order, and why is it important?
Bond order describes the number of chemical bonds between two atoms. It is crucial for understanding molecular structure, stability, and reactivity.
Question 2: How is bond order calculated for CN–?
The bond order of CN– can be calculated using various methods, such as molecular orbital theory, valence bond theory, or experimental techniques like X-ray crystallography.
Question 3: What factors influence the bond order of CN–?
Factors affecting the bond order of CN– include the number of valence electrons, hybridization of atomic orbitals, and resonance.
Question 4: What is the significance of resonance in determining the bond order of CN–?
Resonance, the delocalization of electrons across multiple Lewis structures, affects the bond order of CN– by reducing the localization of the bond and increasing its stability.
Question 5: How does bond order relate to bond length and bond strength?
Bond order is inversely proportional to bond length and directly proportional to bond strength. Shorter bonds with higher bond orders indicate stronger bonds, and vice versa.
Question 6: What are the applications of understanding bond order in chemistry?
Comprehending bond order is crucial in various fields, including materials science, drug design, and catalysis, as it provides insights into molecular properties and reactivity.
These FAQs provide a concise overview of the essential concepts related to calculating the bond order of CN–. For further exploration, the next section delves into advanced topics and applications of bond order calculations.
Tips for Calculating Bond Order of CN–
To enhance your understanding and accuracy when calculating the bond order of CN–, consider these valuable tips:
Tip 1: Utilize Multiple Methods
Employ various techniques like molecular orbital theory and valence bond theory to cross-check your results, ensuring more reliable bond order calculations.
Tip 2: Consider Resonance
Don’t overlook the impact of resonance, as it can alter the bond order by delocalizing electrons. Analyze resonance structures to gain a comprehensive understanding of the bonding.
Tip 3: Examine Bond Length and Strength
Relate bond order to bond length and strength. Shorter bonds with higher bond orders indicate stronger bonds, providing additional insights into the nature of the CN– bond.
Tip 4: Understand Hybridization
Recognize the role of hybridization in shaping the atomic orbitals involved in bonding. Hybridization influences the overlap and symmetry of orbitals, affecting the bond order.
Tip 5: Utilize Quantum Mechanics
Embrace quantum mechanics to delve deeper into the electronic structure and bonding of CN–. This approach provides a rigorous framework for understanding the wave-particle duality and quantum effects influencing bond order.
By incorporating these tips into your approach, you can refine your bond order calculations, gain a deeper understanding of CN– bonding, and expand your knowledge in quantum chemistry.
These tips lay the groundwork for exploring the advanced applications of bond order calculations, enabling you to tackle complex chemical problems with confidence.
Conclusion
In summary, calculating the bond order of CN– involves a comprehensive understanding of atomic orbitals, molecular symmetry, electron configuration, hybridization, resonance, bond length, bond strength, molecular orbital theory, valence bond theory, and quantum mechanics. These concepts provide a holistic framework for determining the number of chemical bonds between carbon and nitrogen in the cyanide ion.
Key points to remember include:
- Bond order is crucial for comprehending molecular structure, stability, and reactivity.
- Resonance delocalizes electrons, influencing bond order and molecular properties.
- Advanced methods, such as quantum mechanics, offer deep insights into the electronic structure and bonding of CN–.
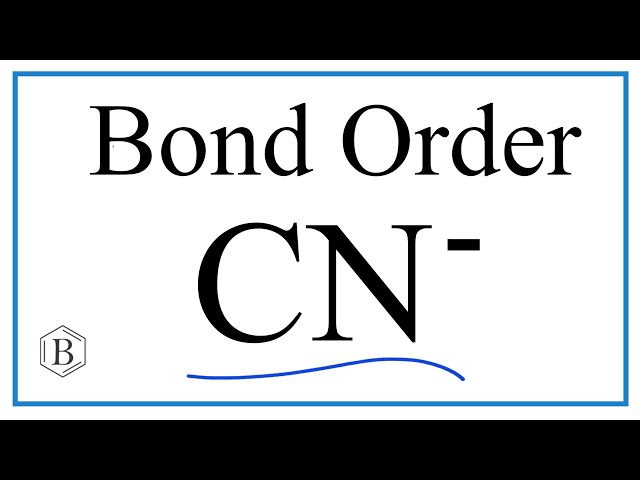