How to Calculate Bond Order: Mastering the Fundamentals of Chemical Bonding
Determining bond order, a crucial aspect of Advanced Placement (AP) Chemistry, enables chemists to comprehend the strength and stability of chemical bonds. In molecules like ethene (C2H4), where carbon atoms share double bonds, understanding bond order is essential for comprehending molecular properties and reactivity.
Calculating bond order provides invaluable insights into molecular structure and behavior. It plays a pivotal role in fields ranging from materials science to biochemistry, where understanding bond strength and stability is paramount. The concept of bond order has its roots in the groundbreaking work of Linus Pauling, who expanded the valence bond theory to include the concept of resonance and hybridization.
This article delves into the intricacies of calculating bond order, equipping readers with a step-by-step guide to determine bond order for various molecular species. By elucidating fundamental concepts, practical applications, and historical context, this comprehensive guide empowers students and professionals alike to confidently navigate the challenges of AP Chemistry and beyond.
How to Calculate Bond Order AP Chem
Understanding how to calculate bond order is crucial for comprehending the strength and stability of chemical bonds. Key aspects to consider include:
- Atomic orbitals
- Valence electrons
- Bonding pairs
- Non-bonding pairs
- Resonance structures
- Molecular geometry
- Bond length
- Bond strength
- Magnetic properties
These aspects are interconnected and provide a comprehensive understanding of bond order. For instance, the number of bonding pairs between atoms determines the bond order, which in turn influences the bond length and strength. Resonance structures contribute to the overall bond order by delocalizing electrons, affecting molecular geometry and magnetic properties. By considering these aspects, chemists can accurately calculate bond order and gain insights into the behavior and reactivity of molecules.
Atomic Orbitals
Atomic orbitals, the foundation of chemical bonding, play a pivotal role in calculating bond order. Each orbital represents a region of space where an electron is likely to be found. Understanding atomic orbitals is essential for comprehending the properties and behavior of molecules.
- Shapes and Symmetry
Atomic orbitals have distinct shapes and symmetries, which determine their overlap and bonding capabilities. - Types of Orbitals
The three main types of atomic orbitals are s, p, and d orbitals, each with unique shapes and energy levels. - Electron Configuration
The electron configuration of an atom, which describes the arrangement of electrons in its orbitals, influences its bonding behavior. - Hybridization
Atomic orbitals can hybridize, combining to form new orbitals with different shapes and properties, impacting bond formation and molecular geometry.
In summary, atomic orbitals provide a framework for understanding the electronic structure of atoms and molecules. By considering the shapes, types, electron configurations, and hybridization of atomic orbitals, chemists can accurately calculate bond order and gain insights into the behavior and reactivity of chemical species.
Valence Electrons
In the realm of chemistry, valence electrons play a central role in determining the bonding behavior of atoms and molecules. They are the electrons that occupy the outermost energy levels of an atom, and their arrangement dictates the atom’s chemical properties and reactivity. Understanding valence electrons is critical for accurately calculating bond order, a fundamental concept in chemistry.
The bond order between two atoms is directly related to the number of valence electrons involved in the bond. A higher bond order indicates a stronger bond and a shorter bond length. Valence electrons participate in chemical bonding by forming covalent bonds, where electrons are shared between atoms to achieve a more stable electron configuration. The number of valence electrons available for bonding determines the maximum bond order that can be formed.
For example, in the case of a carbon-carbon bond, each carbon atom contributes two valence electrons to the bond, resulting in a bond order of 2, which corresponds to a double bond. In contrast, a carbon-hydrogen bond involves the sharing of one valence electron from carbon and one valence electron from hydrogen, resulting in a bond order of 1, indicating a single bond.
Understanding the relationship between valence electrons and bond order is essential for predicting the stability and reactivity of molecules. It enables chemists to design and synthesize new materials with tailored properties and applications. Furthermore, it provides insights into the behavior of molecules in various chemical reactions, guiding the development of new drugs, catalysts, and other functional materials.
Bonding pairs
Bonding pairs are central to calculating bond order in AP Chemistry, representing the electron pairs shared between atoms that form covalent bonds. Understanding bonding pairs is critical for determining the stability and properties of molecules.
- Types of Bonding Pairs
Bonding pairs can be categorized as sigma () or pi () bonds, depending on their orbital overlap and symmetry. - Bond Order Determination
The number of bonding pairs between two atoms directly determines the bond order, which is a measure of bond strength. - Molecular Geometry
Bonding pairs influence the molecular geometry by defining the spatial arrangement of atoms around a central atom. - Bond Properties
Bonding pairs affect bond length, bond strength, and other physical and chemical properties of molecules.
In summary, bonding pairs provide a fundamental framework for understanding and calculating bond order in AP Chemistry. By considering the types, role in bond order determination, impact on molecular geometry, and influence on bond properties, chemists can gain deeper insights into the structure, stability, and reactivity of chemical compounds.
Non-bonding pairs
Non-bonding pairs, also known as lone pairs, are crucial in calculating bond order AP Chem because they represent electron pairs that are not involved in covalent bonding. Understanding their role is essential in determining molecular structure, stability, and reactivity.
Non-bonding pairs occupy atomic orbitals and do not participate in bond formation. However, they influence the overall electron density around an atom, affecting the bond order of adjacent bonds. For instance, in a molecule with a central atom surrounded by both bonding and non-bonding pairs, the non-bonding pairs exert a repulsive force on the bonding pairs, causing them to be oriented away from the non-bonding pairs. This effect can alter the molecular geometry and bond angles.
Calculating bond order considering non-bonding pairs provides insights into molecular properties and behavior. For example, in the case of the water molecule (H2O), the oxygen atom has two non-bonding pairs that repel the two O-H bonds, resulting in a bent molecular geometry and an altered bond order compared to a molecule without non-bonding pairs. This understanding is critical in predicting molecular polarity, reactivity, and intermolecular interactions.
In summary, non-bonding pairs play a significant role in bond order calculations, as they influence the electron density distribution and molecular geometry. Considering non-bonding pairs enables chemists to accurately determine bond orders, predict molecular properties, and gain insights into the behavior and reactivity of chemical compounds.
Resonance structures
In the realm of chemistry, resonance structures play a pivotal role in understanding and calculating bond order, a fundamental concept in AP Chemistry. Resonance structures are hypothetical Lewis structures that represent the delocalization of electrons within a molecule or polyatomic ion. This delocalization results from the presence of multiple possible arrangements of double bonds and lone pairs, which contribute to the overall bonding and stability of the molecule.
Understanding resonance structures is crucial for accurately calculating bond order, as they can affect the number of bonding and non-bonding pairs involved in the bond. For instance, in the case of the benzene molecule (C6H6), resonance structures show that the double bonds are not fixed between specific carbon atoms but are delocalized around the ring. This delocalization leads to an equal distribution of bond order among all carbon-carbon bonds, making them equivalent in length and strength.
The concept of resonance structures has practical applications in various fields of chemistry, including organic chemistry, inorganic chemistry, and biochemistry. By considering resonance structures, chemists can better predict the stability, reactivity, and properties of molecules. This understanding aids in the design and synthesis of new materials, drugs, and functional compounds with tailored properties.
Molecular geometry
Molecular geometry, the three-dimensional arrangement of atoms within a molecule, plays a crucial role in determining bond order through its influence on orbital overlap. The shape of a molecule, dictated by its molecular geometry, affects the spatial orientation of atomic orbitals, which in turn influences the degree of overlap between them. Greater orbital overlap leads to stronger bonds and higher bond order. For example, in a linear molecule with sp orbitals, the head-to-head overlap results in a bond order of 1, whereas in a trigonal planar molecule with sp2 orbitals, the 120 bond angles allow for greater overlap, resulting in a bond order of 1.5.
Conversely, bond order can also impact molecular geometry. Double bonds, with a bond order of 2, typically involve sp2 hybridization, which results in a trigonal planar molecular geometry. Triple bonds, with a bond order of 3, involve sp hybridization, leading to a linear molecular geometry. Thus, calculating bond order not only provides insights into the strength and nature of chemical bonds but also aids in predicting the molecular geometry of a compound.
Understanding the interplay between molecular geometry and bond order is essential for predicting molecular properties, reactivity, and behavior. In catalysis, for instance, the active sites of enzymes often rely on specific molecular geometries to achieve optimal bond order and catalytic efficiency. In materials science, manipulating molecular geometry through bond order calculations enables the design of materials with desired electronic and structural properties. Therefore, the connection between molecular geometry and bond order is a fundamental concept in chemistry, providing a powerful tool for understanding and manipulating the behavior of matter at the molecular level.
Bond Length
Bond length, a fundamental property of chemical bonds, plays a pivotal role in determining bond order and understanding the behavior of molecules. It is the distance between the nuclei of two bonded atoms and provides insights into the strength and nature of the bond.
- Atomic Radii
Bond length is influenced by the atomic radii of the bonded atoms. Larger atoms have larger radii, resulting in longer bond lengths. - Bond Order
Bond order is inversely proportional to bond length. Higher bond orders indicate shorter bond lengths due to increased electron density and stronger attractive forces. - Hybridization
The hybridization of atomic orbitals affects bond length. Orbitals with greater overlap, such as sp hybrid orbitals, lead to shorter bond lengths. - Resonance
Resonance structures can contribute to shorter bond lengths by delocalizing electrons and increasing bond order.
Understanding bond length is essential for predicting molecular properties and reactivity. Shorter bond lengths generally indicate stronger bonds, influencing molecular stability, vibrational frequencies, and chemical reactivity. By considering bond length in conjunction with other factors such as bond order and molecular geometry, chemists can gain a comprehensive understanding of chemical bonding and molecular behavior.
Bond strength
In the realm of “how to calculate bond order ap chem,” understanding bond strength is crucial. It measures the strength of the attractive forces holding atoms together and influences the stability, reactivity, and properties of molecules.
- Bond dissociation energy
The energy required to break a bond, providing a direct measure of bond strength. Higher bond dissociation energies indicate stronger bonds.
- Bond length
A shorter bond length often corresponds to a stronger bond due to increased electron density and stronger attractive forces.
- Bond order
As bond order increases, bond strength generally increases. Higher bond orders indicate more bonding pairs and stronger attractive forces.
- Resonance
Resonance structures can increase bond strength by delocalizing electrons and distributing the bond order over multiple bonds.
Understanding bond strength is essential for predicting the reactivity and behavior of molecules. Stronger bonds lead to more stable molecules, while weaker bonds facilitate bond breaking and the formation of new bonds. Considering bond strength in conjunction with bond order and other molecular properties provides a comprehensive view of chemical bonding and its implications in various chemical processes.
Magnetic Properties
Magnetic properties, arising from the motion of electrons, play a crucial role in understanding and calculating bond order in AP Chemistry. Electron spin, a fundamental property, contributes to the magnetic behavior of molecules and provides valuable insights into their electronic structure.
When electrons pair in molecular orbitals, their spins can either be aligned (parallel) or opposed (antiparallel). The alignment of electron spins influences the magnetic properties of the molecule. Molecules with unpaired electrons, such as oxygen (O2), exhibit paramagnetism, while molecules with all electrons paired, like carbon dioxide (CO2), are diamagnetic. The number of unpaired electrons can be directly related to the bond order of the molecule.
For example, in the case of oxygen (O2), each oxygen atom contributes one unpaired electron to the bond, resulting in a bond order of 2 and paramagnetic behavior. In contrast, in carbon dioxide (CO2), the carbon atom has all its electrons paired, and each oxygen atom contributes two electrons to form double bonds, resulting in a bond order of 2 and diamagnetic behavior. Understanding the relationship between magnetic properties and bond order allows chemists to predict the magnetic behavior of molecules and gain insights into their electronic structures.
Frequently Asked Questions on Bond Order in AP Chemistry
This section addresses frequently asked questions and clarifies common misconceptions regarding bond order calculations in AP Chemistry.
Question 1: What is bond order and why is it important?
Answer: Bond order represents the number of chemical bonds between two atoms, providing insights into bond strength and molecular stability. Accurately determining bond order is crucial for understanding chemical bonding and predicting molecular properties.
Question 2: How do I calculate bond order using the Lewis structure?
Answer: Bond order can be calculated by counting the number of bonding pairs (single bonds count as one, double bonds as two, and triple bonds as three) and subtracting the number of non-bonding pairs.
Question 3: What is resonance, and how does it affect bond order?
Answer: Resonance occurs when a molecule has multiple valid Lewis structures. It delocalizes electrons, affecting bond order by distributing it over multiple bonds.
Question 4: How does hybridization influence bond order?
Answer: Hybridization involves mixing atomic orbitals to form new hybrid orbitals with different shapes and energies. Different hybridization types (e.g., sp, sp2, sp3) can impact bond order by altering the overlap between atomic orbitals.
Question 5: What are the limitations of using bond order to predict molecular properties?
Answer: Bond order is a useful tool, but it does not always accurately predict molecular properties. Other factors, such as electronegativity, resonance, and steric effects, can influence molecular behavior.
Question 6: How is bond order related to bond length and bond strength?
Answer: Bond order is inversely proportional to bond length and directly proportional to bond strength. Higher bond orders correspond to shorter bond lengths and stronger bonds.
These FAQs provide a concise overview of key concepts related to bond order calculations. Understanding these concepts is essential for mastering AP Chemistry and gaining a deeper understanding of chemical bonding.
Transition to the next section:
In the following section, we will explore advanced applications of bond order calculations, including its use in predicting molecular properties and designing new materials.
Tips for Calculating Bond Order in AP Chemistry
Mastering bond order calculations is essential for success in AP Chemistry. Here are some detailed tips to guide you:
Tip 1: Understand Atomic Orbitals and Hybridization
Familiarize yourself with the shapes and energies of atomic orbitals. Hybridization concepts help explain the formation of molecular orbitals and bond order.
Tip 2: Identify Bonding and Non-Bonding Electrons
Distinguish between electrons involved in covalent bonds (bonding pairs) and those not involved (non-bonding pairs). This distinction is crucial for accurate bond order calculations.
Tip 3: Utilize Resonance Structures
Consider resonance structures to account for electron delocalization. This can affect bond order by distributing it over multiple bonds.
Tip 4: Pay Attention to Molecular Geometry
Understand how molecular geometry influences bond order. The arrangement of atoms around a central atom affects orbital overlap and bond strength.
Tip 5: Consider Bond Length and Bond Strength
Relate bond order to bond length and strength. Shorter bond lengths and higher bond strengths typically indicate higher bond orders.
Tip 6: Practice with Examples
Regular practice is key. Solve diverse problems involving bond order calculations to enhance your understanding and accuracy.
Tip 7: Utilize Online Resources
Leverage online resources, such as simulations and tutorials, to supplement your learning and reinforce concepts.
Tip 8: Seek Clarification When Needed
Don’t hesitate to ask for help from your teacher or peers if you encounter difficulties. Clarifying concepts will strengthen your understanding.
By following these tips, you can effectively calculate bond order in AP Chemistry, unlocking a deeper comprehension of chemical bonding and molecular behavior.
Transition to the conclusion:
These tips provide a solid foundation for mastering bond order calculations. In the concluding section, we will explore advanced applications of bond order, enabling you to apply this knowledge to predict molecular properties and design innovative materials.
Conclusion
Throughout this article, we have delved into the intricacies of “how to calculate bond order ap chem.” We have explored the fundamental concepts, practical applications, and historical context of bond order, gaining a comprehensive understanding of its significance in chemistry.
Key insights emerged from our exploration:
- Bond order is a crucial parameter for comprehending the strength and stability of chemical bonds.
- Accurately calculating bond order requires a thorough understanding of atomic orbitals, valence electrons, and resonance structures.
- Bond order is interconnected with molecular geometry, bond length, and bond strength, providing valuable insights into molecular properties and behavior.
Mastering bond order calculations empowers chemists to predict molecular properties, design new materials, and understand the behavior of molecules in various chemical reactions. By unraveling the mysteries of bond order, we unlock the potential for scientific advancements and technological innovations.
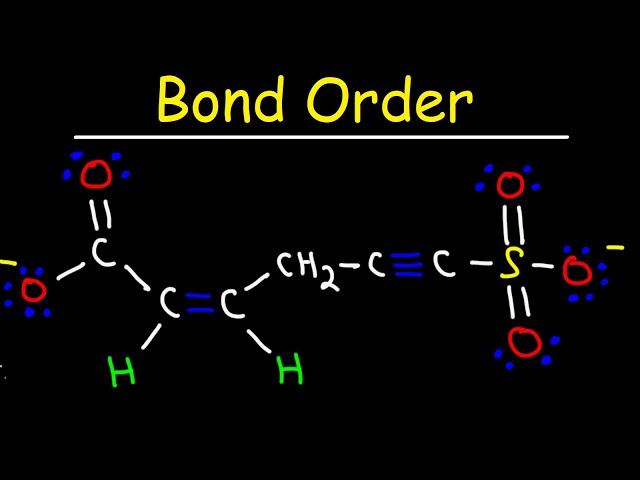