Bond Order Molecular Orbital Theory, often referred to as “how to calculate bond order molecular orbital theory”, is a computational quantum chemistry technique used to calculate the bond order of a molecule. Bond order reflects the strength of a chemical bond between two atoms, and understanding it is vital for predicting molecular properties and chemical reactivity.
This technique is particularly relevant in fields such as organic chemistry, inorganic chemistry, and materials science. By providing insights into bond strength and stability, it helps researchers design new materials with tailored properties, understand reaction mechanisms, and predict the behavior of molecules in various environments. Historically, the development of molecular orbital theory in the 1930s laid the foundation for this technique.
In this article, we will delve into the step-by-step calculation of bond order using molecular orbital theory, exploring its applications and providing practical examples to deepen your understanding of this fundamental chemical concept.
How to Calculate Bond Order Molecular Orbital Theory
Understanding the essential aspects of bond order molecular orbital theory is crucial for accurately calculating bond order and gaining insights into molecular properties and chemical reactivity.
- Molecular Orbitals
- Linear Combination of Atomic Orbitals
- Bonding and Antibonding Orbitals
- Electron Density
- Bond Order
- Resonance Structures
- Hybridization
- Delocalization
These aspects are interconnected and provide a comprehensive framework for understanding the electronic structure and bonding in molecules. By considering the interplay between atomic orbitals, molecular orbitals, and electron density, we can determine the strength and nature of chemical bonds, predict molecular properties, and rationalize chemical reactivity. For instance, the concept of resonance structures helps us understand the delocalization of electrons in certain molecules, leading to unique bonding characteristics and properties.
Molecular Orbitals
Molecular orbitals (MOs) are a fundamental concept in quantum chemistry and play a critical role in understanding the electronic structure and bonding in molecules. They are formed by the linear combination of atomic orbitals (AOs), which are the orbitals of individual atoms. The combination of AOs leads to the formation of MOs that can be bonding, antibonding, or non-bonding in nature.
In the context of bond order molecular orbital theory, MOs are essential for calculating bond order. Bond order is a measure of the strength of a chemical bond between two atoms and is directly related to the number of electrons occupying bonding and antibonding MOs. The greater the number of electrons in bonding MOs and the fewer in antibonding MOs, the stronger the bond order and the stronger the chemical bond. For example, in the hydrogen molecule (H2), the two atomic orbitals (1s orbitals) combine to form two molecular orbitals: a bonding MO (1s) and an antibonding MO (*1s). The two electrons in the H2 molecule occupy the bonding MO, resulting in a bond order of 1, which corresponds to a single bond.
MOs also provide insights into the properties and reactivity of molecules. The energy levels of MOs are related to the stability and reactivity of the molecule. Higher energy MOs are more reactive and can participate in chemical reactions more readily. The symmetry and shape of MOs can also influence the molecular properties, such as polarity, magnetic susceptibility, and spectroscopic properties. Understanding MOs is therefore essential for predicting and explaining the behavior of molecules in various chemical and physical processes.
Linear Combination of Atomic Orbitals
Linear combination of atomic orbitals (LCAO) is a fundamental concept in quantum chemistry that plays a critical role in understanding the electronic structure and bonding in molecules. It provides a theoretical framework for constructing molecular orbitals (MOs) from a set of atomic orbitals (AOs). The LCAO approach is a cornerstone of molecular orbital theory and is essential for calculating bond order using molecular orbital theory.
In the context of bond order molecular orbital theory, LCAO is used to determine the wavefunction of each MO. By combining AOs with appropriate coefficients, the MOs can be expressed as a linear combination of the AOs. The coefficients are determined by solving the Schrdinger equation for the molecule. Once the MOs are known, the bond order can be calculated using the number of electrons occupying the bonding and antibonding MOs.
For example, in the hydrogen molecule (H2), the two 1s AOs combine to form two MOs: a bonding MO (1s) and an antibonding MO (*1s). The bonding MO is formed by the in-phase combination of the two 1s AOs, while the antibonding MO is formed by the out-of-phase combination. The two electrons in the H2 molecule occupy the bonding MO, resulting in a bond order of 1, which corresponds to a single bond.
LCAO is also essential for understanding the properties and reactivity of molecules. The energy levels of the MOs are determined by the coefficients of the AOs in the LCAO combination. Higher energy MOs are more reactive and can participate in chemical reactions more readily. The symmetry and shape of the MOs can also influence the molecular properties, such as polarity, magnetic susceptibility, and spectroscopic properties. Understanding LCAO is therefore essential for predicting and explaining the behavior of molecules in various chemical and physical processes.
Bonding and Antibonding Orbitals
In the context of “how to calculate bond order molecular orbital theory”, understanding bonding and antibonding orbitals is crucial. These orbitals arise from the linear combination of atomic orbitals (LCAO) and play a significant role in determining the strength and nature of chemical bonds. Bonding orbitals are formed when atomic orbitals overlap in-phase, leading to constructive interference and lower energy. Antibonding orbitals, on the other hand, result from out-of-phase overlap, causing destructive interference and higher energy.
The number of electrons occupying bonding and antibonding orbitals directly affects the bond order, a measure of bond strength. Bonding orbitals accommodate electrons that contribute to the overall stability of the molecule, while antibonding orbitals contain electrons that weaken the bond. For instance, in the hydrogen molecule (H2), the two 1s atomic orbitals combine to form a bonding molecular orbital (1s) and an antibonding molecular orbital (*1s). The two electrons in H2 occupy the bonding orbital, resulting in a bond order of 1, indicating a stable single bond.
The concept of bonding and antibonding orbitals finds practical applications in various fields. In inorganic chemistry, it helps explain the bonding and properties of coordination complexes. In organic chemistry, it provides insights into the reactivity and stability of organic molecules, guiding the design of new compounds with tailored properties. Understanding these orbitals is also essential for interpreting spectroscopic data, such as ultraviolet-visible (UV-Vis) and nuclear magnetic resonance (NMR) spectra, which provide valuable information about molecular structure and dynamics.
Electron Density
Electron density plays a pivotal role in “how to calculate bond order molecular orbital theory” as it determines the probability of finding an electron in a given region of space. The electron density distribution around atoms and molecules provides crucial insights into chemical bonding and molecular properties.
In the context of molecular orbital theory, electron density is directly related to the wavefunction of the molecule. The wavefunction, which describes the quantum state of the molecule, determines the probability of finding electrons in different regions of space. By calculating the electron density, we can visualize the distribution of electrons and understand how they are delocalized over the molecule.
One practical application of electron density analysis is in the study of chemical bonding. By analyzing the electron density distribution, we can identify bonding and antibonding regions within a molecule. Bonding regions, where the electron density is high, indicate areas of constructive interference between atomic orbitals, leading to the formation of strong chemical bonds. Conversely, antibonding regions, characterized by low electron density, show regions of destructive interference and weaker bonds or even electron repulsion.
Furthermore, electron density analysis is essential for understanding molecular properties such as polarity, reactivity, and spectroscopic behavior. The distribution of electron density can provide insights into the molecular dipole moment, which determines the polarity of the molecule. The electron density around specific atoms or groups can also indicate regions of high or low reactivity, guiding the design of new molecules with tailored properties. Additionally, electron density analysis can aid in the interpretation of spectroscopic data, such as X-ray diffraction and nuclear magnetic resonance (NMR), providing valuable information about molecular structure and dynamics.
Bond Order
In the realm of “how to calculate bond order molecular orbital theory,” the concept of “Bond Order” holds immense significance. It provides a quantitative measure of the strength and nature of the chemical bond between two atoms within a molecule and serves as a cornerstone for understanding molecular structure and reactivity.
- Bond Length
Bond Order is inversely related to Bond Length. Shorter bond lengths indicate stronger bonds and higher Bond Orders, reflecting a greater overlap between atomic orbitals.
- Bond Energy
Bond Order directly correlates with Bond Energy. Stronger bonds, characterized by higher Bond Orders, require more energy to break.
- Bond Type
Bond Order helps distinguish between different types of bonds. For instance, a Bond Order of 1 represents a single bond, 2 corresponds to a double bond, and 3 indicates a triple bond.
- Resonance Structures
In molecules with resonance structures, Bond Order provides insights into the delocalization of electrons and the resonance hybrid.
By understanding these facets of Bond Order, scientists can gain deeper insights into the electronic structure, stability, and reactivity of molecules. It allows chemists to predict and explain a wide range of chemical phenomena, from molecular spectroscopy to reaction mechanisms.
Resonance Structures
Resonance Structures play a crucial role in “how to calculate bond order molecular orbital theory” by providing a theoretical framework for understanding the delocalization of electrons within a molecule. Resonance structures are different Lewis structures that represent the same molecule but vary in the placement of double bonds and lone pairs. This concept is particularly important in molecules with alternating single and double bonds, such as benzene.
In the context of bond order molecular orbital theory, resonance structures help us determine the average bond order of each bond in the molecule. By considering all possible resonance structures and calculating the bond order for each structure, we can obtain a more accurate representation of the electron distribution and bonding within the molecule. This is particularly useful for understanding the stability and reactivity of molecules, as resonance structures can indicate areas of high electron density and potential reactivity.
A real-life example of resonance structures and bond order molecular orbital theory can be seen in the benzene molecule. Benzene has two resonance structures, each with alternating single and double bonds. By considering both resonance structures and calculating the bond order for each C-C bond, we find that the bond order is 1.5. This indicates that the C-C bonds in benzene are stronger than typical single bonds but weaker than typical double bonds, reflecting the delocalization of electrons within the benzene ring.
Understanding the connection between resonance structures and bond order molecular orbital theory has practical applications in various fields of chemistry, including organic chemistry, inorganic chemistry, and biochemistry. By considering resonance structures, chemists can better predict the stability, reactivity, and properties of molecules, aiding in the design and synthesis of new materials and pharmaceuticals.
Hybridization
Hybridization is a fundamental concept in chemistry that plays a vital role in “how to calculate bond order molecular orbital theory.” It describes the process of combining atomic orbitals to form new hybrid orbitals with different shapes and energies. This hybridization is crucial for understanding the electronic structure, bonding, and properties of molecules.
In the context of bond order molecular orbital theory, hybridization determines the type and strength of chemical bonds formed between atoms. By considering the hybridized orbitals of the bonded atoms, we can predict the geometry, bond angles, and bond order of the molecule. For example, in the case of methane (CH4), the carbon atom undergoes sp3 hybridization, resulting in four equivalent hybrid orbitals that form tetrahedral bonds with hydrogen atoms. This hybridization leads to a bond order of 1 for each C-H bond and explains the tetrahedral geometry of the molecule.
Hybridization also provides insights into the reactivity and stability of molecules. Molecules with stronger bonds, formed by orbitals with greater overlap, are generally more stable and less reactive. Understanding hybridization allows chemists to design and synthesize molecules with tailored properties by controlling the hybridization of the constituent atoms. This knowledge finds applications in various fields, including organic chemistry, inorganic chemistry, and materials science.
In summary, hybridization is an essential component of “how to calculate bond order molecular orbital theory.” By considering the hybridization of atoms, we can accurately predict the electronic structure, bonding, and properties of molecules. This understanding has practical significance in chemistry, enabling the design and synthesis of new materials with tailored properties and reactivities.
Delocalization
Delocalization, in the context of “how to calculate bond order molecular orbital theory,” refers to the distribution of electrons over multiple atoms or molecules, rather than being confined to a specific location. This phenomenon plays a crucial role in determining the electronic structure and properties of molecules.
Delocalization arises when the molecular orbitals formed by the combination of atomic orbitals extend over several atoms. This often occurs when there are overlapping p-orbitals or when there is resonance between multiple Lewis structures. The delocalization of electrons leads to a decrease in the overall energy of the molecule, making it more stable. It also affects the bond order, as delocalized electrons contribute to the bonding between multiple atoms.
A classic example of delocalization is benzene, a molecule with a ring of six carbon atoms. In benzene, the p-orbitals of the carbon atoms overlap to form a continuous ring of delocalized electrons. This delocalization results in a bond order of 1.5 for each carbon-carbon bond, indicating a higher degree of bonding than would be expected for a single bond.
Understanding delocalization is crucial for accurately calculating bond order using molecular orbital theory. By considering the delocalization of electrons, we can determine the average bond order for each bond in the molecule, which provides insights into the stability and reactivity of the molecule. This understanding finds applications in various fields of chemistry, including organic chemistry, inorganic chemistry, and materials science.
Frequently Asked Questions about Bond Order Molecular Orbital Theory
This section addresses common questions and clarifies concepts related to “how to calculate bond order molecular orbital theory.” The FAQs provide concise answers to anticipated queries, fostering a deeper understanding of the subject.
Question 1: What is bond order?
Bond order is a quantitative measure of the strength of a chemical bond between two atoms. It is calculated using molecular orbital theory and provides insights into the stability and reactivity of molecules.
Question 2: How do I calculate bond order using molecular orbital theory?
To calculate bond order, you need to determine the molecular orbitals involved in the bond and the number of electrons occupying those orbitals. The bond order is half the difference between the number of bonding and antibonding electrons.
Question 3: What is the relationship between bond order and bond length?
Bond order is inversely proportional to bond length. Stronger bonds have a higher bond order and a shorter bond length, while weaker bonds have a lower bond order and a longer bond length.
Question 4: How does hybridization affect bond order?
Hybridization influences bond order by determining the type and strength of the atomic orbitals involved in bonding. Different hybridization schemes result in different bond orders, affecting the properties and reactivity of molecules.
Question 5: What is delocalization, and how does it impact bond order?
Delocalization refers to the distribution of electrons over multiple atoms or molecules. It can lead to resonance and the formation of delocalized molecular orbitals. Delocalization affects bond order by altering the electron distribution and bond strengths.
Question 6: Why is bond order important in chemistry?
Bond order is a fundamental concept in chemistry as it provides a quantitative understanding of chemical bonding. It helps predict molecular properties, explain chemical reactivity, and design new materials with tailored properties.
These FAQs provide a concise overview of key aspects related to “how to calculate bond order molecular orbital theory.” Understanding these concepts is crucial for gaining a deeper insight into the electronic structure and properties of molecules.
In the following section, we will explore practical applications of bond order molecular orbital theory in various fields of chemistry, showcasing its significance in real-world scenarios.
Tips for Calculating Bond Order Using Molecular Orbital Theory
To effectively calculate bond order using molecular orbital theory, consider the following practical tips:
Tip 1: Understand Molecular Orbital Theory Fundamentals
Grasp the concepts of atomic orbitals, molecular orbitals, and electron density to lay a strong foundation for bond order calculations.
Tip 2: Determine the Molecular Orbitals Involved
Identify the atomic orbitals that combine to form the molecular orbitals participating in the bond of interest.
Tip 3: Calculate the Number of Bonding and Antibonding Electrons
Count the electrons occupying the bonding and antibonding molecular orbitals to determine their respective populations.
Tip 4: Consider Resonance Structures
If the molecule exhibits resonance, account for all contributing resonance structures to obtain an accurate representation of electron distribution.
Tip 5: Utilize Computational Tools
Employ computational chemistry software or online tools to simplify and expedite bond order calculations.
Tip 6: Understand the Impact of Hybridization
Recognize how hybridization influences the type and strength of atomic orbitals involved in bonding, affecting the bond order.
Tip 7: Consider Delocalization Effects
Account for the delocalization of electrons over multiple atoms, as it can alter bond order and molecular properties.
Tip 8: Interpret Bond Order in Context
Relate the calculated bond order to the molecule’s stability, reactivity, and other chemical properties.
These tips provide a roadmap for effectively calculating bond order using molecular orbital theory. By following these guidelines, you can gain valuable insights into the electronic structure and bonding characteristics of molecules.
In the concluding section, we will delve into the broader applications of bond order molecular orbital theory, showcasing its utility in various fields of chemistry and its importance in advancing our understanding of molecular systems.
Conclusion
This comprehensive exploration of “how to calculate bond order molecular orbital theory” has provided valuable insights into the electronic structure and bonding characteristics of molecules. Key concepts such as molecular orbitals, electron density, and resonance structures have been explored, emphasizing their significance in determining bond order.
The article highlights the interplay between atomic orbitals, molecular orbitals, and electron distribution in shaping bond order. By understanding how to calculate bond order using molecular orbital theory, chemists can gain a deeper appreciation of molecular stability, reactivity, and various properties. This knowledge empowers researchers to design new materials with tailored properties and predict the behavior of molecules in diverse chemical and biological systems.
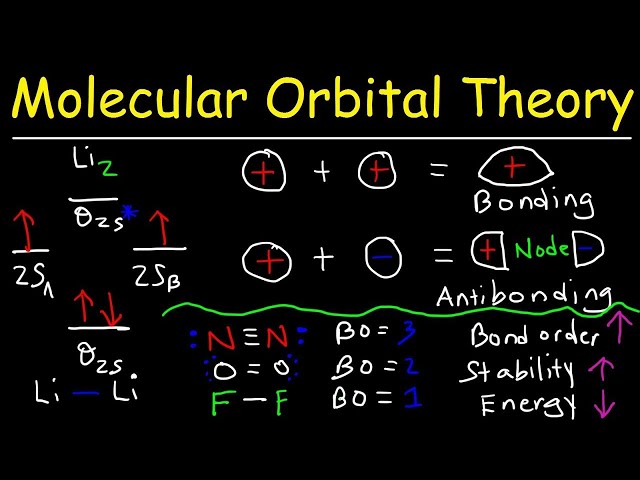