Calculating bond order and bond length, essential in chemistry, determines the strength and stability of chemical bonds.
Understanding these properties enables chemists to predict molecular structure, reactivity, and properties of various materials. A key milestone was Linus Pauling’s development of valence bond theory, which provided foundational insights into bond formation.
This article will explore the methods for calculating bond order and bond length, highlighting their significance and providing practical examples.
How to Calculate Bond Order and Bond Length
Understanding bond order and bond length is essential in chemistry, as they provide insights into the strength and stability of chemical bonds. These properties influence molecular structure, reactivity, and material properties.
- Bond Order: Measure of bond strength
- Bond Length: Distance between bonded atoms
- Resonance: Delocalization of electrons
- Hybridization: Mixing of atomic orbitals
- Electronegativity: Attraction for electrons
- Atomic Radius: Size of atoms
- Bond Type: Single, double, or triple bond
- Molecular Orbital Theory: Quantum mechanical model of bonding
- Valence Bond Theory: Classical model of bonding
Calculating bond order and bond length involves considering these aspects. Resonance, hybridization, and electronegativity influence bond order, while atomic radius, bond type, and molecular orbital theory impact bond length. Understanding these interconnections enables chemists to predict and analyze molecular structures and properties.
Bond Order
Bond order, a measure of bond strength, plays a critical role in calculating bond order and bond length. It reflects the number of electron pairs shared between two atoms, indicating the strength and stability of their chemical bond.
Calculating bond order involves determining the number of bonding and antibonding electrons. Bonding electrons contribute to the bond’s strength, while antibonding electrons weaken it. By considering the molecular orbital interactions and electron configurations, chemists can calculate bond order and predict bond strength.
Bond order directly influences bond length. A higher bond order typically corresponds to a shorter bond length due to increased electron sharing and stronger electrostatic attraction between the atoms. This relationship is crucial in understanding molecular geometry, stability, and reactivity. For example, double bonds, with a bond order of 2, are shorter and stronger than single bonds, which have a bond order of 1.
Understanding bond order and bond length enables chemists to predict and analyze the properties of chemical compounds. This knowledge finds applications in various fields, including materials science, drug design, and catalysis. By manipulating bond order and bond length, scientists can tailor materials with specific properties and design molecules with desired reactivities.
Bond Length
In calculating bond order and bond length, understanding the concept of bond length is crucial. Bond length refers to the distance between the nuclei of two bonded atoms and provides valuable insights about the strength and nature of the bond.
- Atomic Radii: The size of the atoms involved directly influences bond length. Larger atoms generally form longer bonds.
- Bond Order: As discussed earlier, bond order is directly related to bond length. Higher bond orders typically correspond to shorter bond lengths.
- Hybridization: The hybridization of atomic orbitals also affects bond length. Hybrid orbitals with more s-character tend to form shorter bonds.
- Electronegativity: The electronegativity difference between the bonded atoms can influence bond length. A greater electronegativity difference leads to a shorter bond length due to stronger electrostatic attraction.
Understanding bond length is essential in predicting molecular geometry, stability, and reactivity. By considering the factors that influence bond length, chemists can gain valuable insights into the behavior and properties of chemical compounds.
Resonance
Resonance, a fundamental concept in chemistry, plays a crucial role in calculating bond order and bond length. It arises when multiple Lewis structures can be drawn for a molecule, indicating the delocalization of electrons across several atoms.
- Electron Delocalization: Electrons are not confined to specific bonds but spread over multiple atoms, affecting bond order and length.
- Resonance Structures: Different Lewis structures represent the same molecule, each contributing to the resonance hybrid.
- Bond Order and Length: Resonance affects bond order and length by distributing electron density differently, leading to an overall equalization of bond lengths and orders.
Understanding resonance is essential for accurately calculating bond order and bond length. It provides a more accurate representation of the molecular structure and bonding, allowing chemists to predict molecular properties and reactivity.
Hybridization
In calculating bond order and bond length, hybridization plays a critical role. Hybridization is the process of mixing atomic orbitals to form new hybrid orbitals with different shapes and energies. This mixing influences the geometry, bonding, and properties of molecules.
Hybridization affects bond order and length by altering the overlap of atomic orbitals. For example, in carbon, the 2s and three 2p orbitals hybridize to form four equivalent sp3 orbitals. These sp3 orbitals have a tetrahedral geometry and overlap more effectively with each other, leading to stronger bonds and shorter bond lengths than would be possible with unhybridized orbitals.
Understanding hybridization is crucial for accurately calculating bond order and bond length. It provides insights into the three-dimensional structure of molecules, their bonding characteristics, and their overall stability. Chemists use hybridization theory to predict molecular geometry, explain bonding patterns, and design new materials with tailored properties.
Electronegativity
Electronegativity, a measure of an atom’s attraction for electrons, significantly impacts bond order and bond length calculations. A higher electronegativity indicates a stronger attraction for electrons, leading to a shift in electron density towards the more electronegative atom.
In covalent bonds, this electronegativity difference between bonded atoms influences bond polarity and bond strength. A greater electronegativity difference results in a more polar bond, where electrons are unequally shared. Consequently, the bond order, a measure of electron pair sharing, decreases. For example, in a bond between carbon and fluorine, fluorine’s higher electronegativity draws electron density towards itself, reducing the bond order compared to a bond between carbon and hydrogen.
Electronegativity also affects bond length. The stronger the electronegative attraction, the shorter the bond length. This is because the more electronegative atom attracts electrons closer to its nucleus, reducing the internuclear distance. Understanding electronegativity is crucial for accurate bond order and bond length calculations, providing insights into molecular structure, reactivity, and properties.
In summary, electronegativity plays a critical role in calculating bond order and bond length. It influences electron distribution, bond polarity, bond order, and bond length, helping chemists understand and predict the behavior of chemical bonds and molecules.
Atomic Radius
Atomic radius, a crucial factor in “how to calculate bond order and bond length,” significantly influences molecular structure and properties. The size of atoms directly affects the distance between bonded atoms, impacting bond length and, consequently, bond order.
Larger atoms possess larger atomic radii, leading to longer bond lengths. This is because the valence electrons in larger atoms are further from the nucleus, resulting in weaker electrostatic attraction between the nuclei of bonded atoms. For example, the bond length between carbon and hydrogen is shorter than that between carbon and iodine due to the smaller atomic radius of hydrogen.
Understanding the relationship between atomic radius and bond length is essential for predicting molecular geometry and stability. In crystal structures, atomic radii determine the packing efficiency and interatomic distances, influencing material properties such as hardness and thermal conductivity. Accurate bond length calculations, considering atomic radii, are crucial for designing materials with tailored properties.
In summary, atomic radius plays a critical role in “how to calculate bond order and bond length,” influencing the distance between bonded atoms and, subsequently, the bond order. This understanding is vital for predicting molecular structures, properties, and the design of materials with specific characteristics.
Bond Type
In “how to calculate bond order and bond length,” understanding bond types is crucial. They determine the number of electron pairs shared between atoms, influencing bond strength and length.
- Bond Order: Single bonds have a bond order of 1, double bonds have a bond order of 2, and triple bonds have a bond order of 3. Bond order is directly related to bond strength, with higher bond orders indicating stronger bonds.
- Bond Length: Bond length is inversely related to bond order. Single bonds are longer than double bonds, and double bonds are longer than triple bonds. This is because the more electron pairs shared between atoms, the shorter and stronger the bond.
- Examples: Ethane (C2H6) contains carbon-carbon single bonds, ethene (C2H4) contains a carbon-carbon double bond, and acetylene (C2H2) contains a carbon-carbon triple bond.
Understanding bond types is essential for accurately calculating bond order and bond length, which are fundamental parameters in predicting molecular structure, stability, and reactivity. Different bond types result in distinct physical and chemical properties, influencing material design and various chemical processes.
Molecular Orbital Theory
Molecular Orbital Theory (MOT), a quantum mechanical model, provides a framework for understanding the electronic structure of molecules. It’s a cornerstone of computational chemistry, used to calculate bond order and bond length, providing insights into the nature of chemical bonds.
MOT describes molecular orbitals as combinations of atomic orbitals, explaining how electrons are distributed within a molecule. By considering the wave functions and energies of these molecular orbitals, chemists can determine the bond order and bond length between atoms.
For instance, in the hydrogen molecule (H2), MOT predicts a bond order of 1 and a bond length of 0.74 . The single bond in H2 results from the overlap of two atomic orbitals, forming a molecular orbital that accommodates both electrons. The bond order of 1 indicates a strong bond, consistent with the short bond length.
Understanding MOT is crucial for accurate bond order and bond length calculations, which are essential for predicting molecular properties, reactivity, and designing new materials. MOT enables chemists to investigate complex molecular systems, such as transition metal complexes and organic polymers, providing valuable insights into their electronic structures and bonding characteristics.
Valence Bond Theory
Valence Bond Theory (VBT), a classical model of bonding, provides an essential framework for understanding the electronic structure of molecules and calculating bond order and bond length. This theory visualizes chemical bonds as the result of the overlap between atomic orbitals, considering factors such as hybridization, resonance, and electronegativity.
- Hybrid Orbitals: VBT explains how atomic orbitals can hybridize to form new orbitals with different shapes and energies. This hybridization influences bond strength and bond angles, affecting bond order and bond length.
- Resonance Structures: VBT recognizes that some molecules can be represented by multiple Lewis structures called resonance structures. These structures contribute to the overall bonding picture, affecting the distribution of electron density and influencing bond order and bond length.
- Electronegativity: VBT considers the electronegativity of atoms, which describes their ability to attract electrons. This electronegativity difference between bonded atoms affects the polarity of the bond and the distribution of electron density, impacting bond order and bond length.
- Bond Order: VBT establishes the concept of bond order, which measures the strength of a chemical bond. Bond order is determined by the number of electron pairs shared between atoms and is directly related to bond length, with higher bond orders corresponding to shorter bond lengths.
By considering these factors, VBT provides a qualitative understanding of bonding in molecules and allows for the estimation of bond order and bond length. This theory serves as a foundation for more advanced quantum mechanical models and is widely used in chemistry to rationalize molecular structures and properties.
FAQs on Calculating Bond Order and Bond Length
This section addresses common questions and misconceptions regarding the calculation of bond order and bond length, providing concise and informative answers.
Question 1: What is the significance of bond order and bond length in chemistry?
Answer: Bond order and bond length are fundamental parameters that provide insights into the strength, stability, and properties of chemical bonds. Bond order indicates the number of electron pairs shared between atoms, while bond length measures the distance between their nuclei. Understanding these properties is crucial for predicting molecular structure, reactivity, and material behavior.
Question 2: How do I calculate bond order?
Answer: Bond order can be calculated using various methods, including the Lewis structure method, the molecular orbital theory method, and the valence bond theory method. Each method considers different factors such as the number of bonding and antibonding electrons, orbital overlap, and hybridization.
Question 3: What factors influence bond length?
Answer: Bond length is primarily influenced by bond order, atomic radii, and electronegativity. Higher bond orders typically correspond to shorter bond lengths, while larger atomic radii and greater electronegativity differences generally lead to longer bond lengths.
Question 4: How does resonance affect bond order and bond length?
Answer: Resonance, which involves the delocalization of electrons across multiple atoms, can affect bond order and bond length. Resonance structures contribute to the overall bonding picture, potentially equalizing bond lengths and orders within the molecule.
Question 5: Why is it important to consider hybridization when calculating bond order and bond length?
Answer: Hybridization, which describes the mixing of atomic orbitals to form new hybrid orbitals, influences the overlap of atomic orbitals and the resulting bond strength and length. Different hybridization schemes can lead to variations in bond order and bond length.
Question 6: How are bond order and bond length used in practice?
Answer: Understanding bond order and bond length is essential in various fields of chemistry, including molecular modeling, materials science, and drug design. These properties are used to predict molecular structures, optimize material properties, and design molecules with desired reactivity and functionality.
These FAQs provide a concise overview of the key concepts and methods involved in calculating bond order and bond length. In the following sections, we will delve deeper into the theoretical background and applications of these properties in chemistry.
Tips for Calculating Bond Order and Bond Length
Understanding how to accurately calculate bond order and bond length is crucial for chemists. These tips provide practical guidance to enhance your understanding and application of these concepts:
Tip 1: Visualize molecular orbitals to understand bond formation.
Tip 2: Consider resonance structures to account for electron delocalization.
Tip 3: Calculate bond order using the molecular orbital theory method for precise results.
Tip 4: Determine bond length by measuring the distance between atomic nuclei.
Tip 5: Understand the relationship between bond order and bond length to predict molecular properties.
Tip 6: Utilize computational tools to simplify complex bond order and bond length calculations.
Tip 7: Consider hybridization and electronegativity when analyzing bond strength and length.
Tip 8: Apply these concepts to predict molecular structures, reactivity, and material properties.
By following these tips, you can effectively calculate bond order and bond length, gaining valuable insights into the behavior and properties of chemical compounds.
In the final section of this article, we will explore advanced applications of these concepts in fields such as materials science and drug design, demonstrating their significance in modern chemistry.
Conclusion
In this article, we have explored the fundamental concepts and methods involved in calculating bond order and bond length. By understanding these properties, chemists can gain insights into the strength, stability, and reactivity of chemical bonds, which are essential for predicting molecular structure and properties.
Key takeaways include the relationship between bond order and bond strength, the influence of resonance and hybridization on bond order and length, and the practical applications of these concepts in chemistry. Understanding how to calculate bond order and bond length empowers chemists to design and optimize materials, predict reaction outcomes, and develop new drugs and technologies. As we continue to unravel the intricacies of chemical bonding, the significance of these concepts will only grow in the years to come.
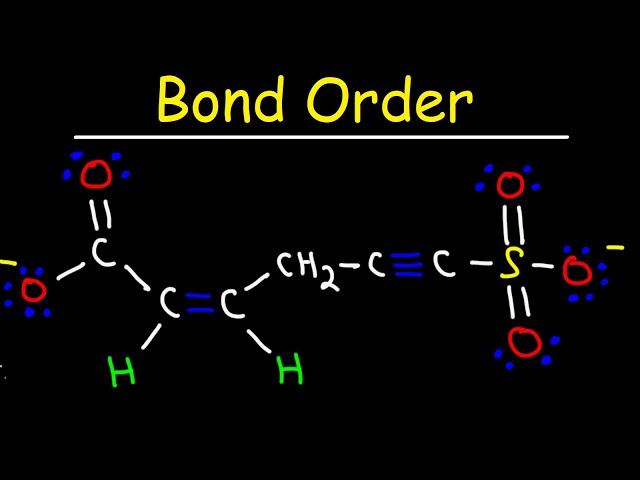