Calculating bond order is a fundamental aspect of understanding chemical bonding. It provides insights into the stability, strength, and properties of chemical bonds.
The concept of bond order has played a significant role in the development of chemical theories, enabling chemists to predict and explain a wide range of chemical phenomena. One notable historical development was the introduction of the valence bond theory by Linus Pauling, which provided a quantum mechanical basis for understanding bond order.
In this article, we will delve into the topic of calculating bond order, exploring the various methods and their applications. We will also discuss the significance of bond order in predicting molecular properties and chemical reactivity.
How to Calculate Bond Order Easily
Calculating bond order accurately is essential for understanding the properties and behavior of chemical compounds. Key aspects to consider include:
- Atomic orbital overlap
- Electron configuration
- Molecular symmetry
- Bond length
- Bond strength
- Bond polarity
- Magnetic properties
- Reactivity
- Spectroscopic properties
- Thermodynamic properties
These aspects are interconnected and provide a comprehensive understanding of bond order. For instance, atomic orbital overlap determines the extent of electron sharing, which influences bond length and strength. Bond polarity, in turn, affects molecular reactivity and spectroscopic properties. By considering these key aspects, chemists can accurately calculate bond order and gain insights into the nature and behavior of chemical bonds.
Atomic Orbital Overlap
Atomic orbital overlap plays a central role in determining bond order. It refers to the extent to which atomic orbitals of adjacent atoms overlap in space, creating a region of high electron density. The degree of overlap influences the strength and properties of the resulting chemical bond.
Greater atomic orbital overlap leads to stronger bonds. This is because the electrons in the overlapping orbitals are more likely to be shared between the atoms, forming a more stable bond. Conversely, less overlap results in weaker bonds.
Calculating bond order takes into account the symmetry and overlap of atomic orbitals. By understanding the relationship between atomic orbital overlap and bond order, chemists can predict the properties and behavior of molecules. For example, in the case of a double bond, there is greater overlap between the atomic orbitals involved, resulting in a higher bond order and stronger bond compared to a single bond.
In summary, atomic orbital overlap is a critical component of bond order calculations. It provides insights into the strength, stability, and properties of chemical bonds, enabling chemists to better understand and predict the behavior of molecules.
Electron Configuration
Electron configuration, referring to the distribution of electrons in atomic orbitals, plays a crucial role in determining bond order. It provides insights into the number and type of bonds an atom can form, as well as the strength and properties of those bonds.
The relationship between electron configuration and bond order is rooted in the Aufbau principle and Hund’s rule. These principles govern the filling of atomic orbitals and dictate the electronic structure of atoms. By understanding the electron configuration of atoms involved in a bond, chemists can predict the number of unpaired electrons and the type of orbitals that will overlap to form the bond.
Consider the example of a carbon atom. With an electron configuration of 1s22s22p2, carbon has two unpaired electrons in its 2p orbitals. These unpaired electrons can overlap with unpaired electrons from other atoms, such as hydrogen or oxygen, to form single or double bonds. By analyzing the electron configurations of the participating atoms, chemists can determine the maximum bond order possible.
Understanding the connection between electron configuration and bond order has practical applications in various fields. It enables chemists to design and synthesize new materials with tailored properties, predict the reactivity of molecules, and understand the behavior of chemical systems in different environments. By harnessing this knowledge, scientists can develop advanced technologies and innovations.
Molecular symmetry
Molecular symmetry plays a pivotal role in determining bond order, offering valuable insights into the electronic structure and properties of molecules. It describes the arrangement of atoms and their corresponding orbitals in a molecule, providing a framework for understanding the distribution of electron density and the formation of chemical bonds.
Molecular symmetry directly influences the overlap of atomic orbitals, which is a critical component of bond order calculations. Symmetrical molecules exhibit specific patterns of orbital overlap, leading to predictable bond orders. For instance, in a linear molecule like carbon dioxide (CO2), the p orbitals of the carbon and oxygen atoms overlap head-to-head, resulting in a double bond between the carbon and each oxygen atom. The high degree of symmetry in CO2 allows for a straightforward determination of bond order based on the molecular geometry.
Understanding molecular symmetry is essential for calculating bond order accurately. It enables chemists to predict the electronic structure, stability, and reactivity of molecules. This knowledge finds practical applications in various fields, including materials science, catalysis, and drug design. By harnessing the principles of molecular symmetry, scientists can design and synthesize novel materials with tailored properties, optimize catalytic processes, and develop targeted therapies for diseases. Comprehending the connection between molecular symmetry and bond order empowers chemists to explore and manipulate the molecular world with greater precision and control.
In summary, molecular symmetry serves as a crucial factor in calculating bond order easily. It provides a systematic approach to understanding atomic orbital overlap, electron distribution, and the properties of chemical bonds. By leveraging this knowledge, chemists gain valuable insights into molecular structure, reactivity, and applications, ultimately contributing to advancements in science and technology.
Bond length
Bond length, defined as the distance between the nuclei of two bonded atoms, is intricately connected to the calculation of bond order. A shorter bond length typically corresponds to a higher bond order, indicating a stronger bond. This relationship arises from the inverse relationship between bond length and bond strength. A shorter bond length implies a greater overlap of atomic orbitals, leading to increased electron sharing and a more robust bond.
For instance, in the case of carbon-carbon bonds, a single bond has a longer bond length (approximately 1.54 ) compared to a double bond (approximately 1.34 ) or a triple bond (approximately 1.20 ). This variation in bond lengths reflects the varying degrees of orbital overlap and bond order. The double bond, with its greater orbital overlap, exhibits a shorter bond length and higher bond order compared to the single bond. Similarly, the triple bond, with its maximum orbital overlap, has the shortest bond length and highest bond order among the three.
Understanding the relationship between bond length and bond order is crucial for various applications. In materials science, it helps researchers design and engineer materials with tailored properties by controlling the bond lengths and, consequently, the bond orders. In chemistry, it enables the prediction of molecular structures, reactivity, and spectroscopic properties. Additionally, in biochemistry, it provides insights into enzyme catalysis and protein folding, where bond lengths and bond orders play a critical role in determining protein structure and function.
Bond strength
Bond strength, a measure of the force required to break a bond between two atoms, is intricately connected to the calculation of bond order. Understanding this relationship is pivotal in chemistry, as it provides insights into the stability, reactivity, and properties of chemical compounds.
Bond strength is directly proportional to bond order. A higher bond order indicates a stronger bond, while a lower bond order corresponds to a weaker bond. This relationship arises from the fact that bond order reflects the number of electron pairs shared between two atoms. The more electron pairs shared, the stronger the bond.
For instance, a carbon-carbon single bond has a bond order of 1, indicating that there is one electron pair shared between the two carbon atoms. This bond is relatively weak and can be easily broken. In contrast, a carbon-carbon double bond has a bond order of 2, indicating that there are two electron pairs shared between the carbon atoms. This bond is significantly stronger than the single bond and requires more energy to break.
Calculating bond order accurately is essential for predicting bond strength and understanding the behavior of molecules. By leveraging this knowledge, chemists can design and synthesize materials with tailored properties, develop new drugs, and engineer chemical reactions more efficiently. Furthermore, this understanding finds applications in diverse fields such as catalysis, materials science, and biochemistry, empowering scientists to explore and manipulate the molecular world with greater precision.
Bond polarity
Bond polarity refers to the uneven distribution of electrons within a covalent bond, resulting in a partial positive charge on one atom and a partial negative charge on the other. This polarity arises from differences in electronegativity, which is the ability of an atom to attract electrons towards itself. A more electronegative atom will have a greater pull on the shared electrons, creating a polar covalent bond.
Understanding bond polarity is a critical component of calculating bond order easily. Bond order, which represents the number of electron pairs shared between two atoms, is directly influenced by the polarity of the bond. In a polar covalent bond, the electron pair is not shared equally, leading to a fractional bond order. The extent of bond polarity affects the overall bond order, and considering this polarity is essential for accurate bond order calculations.
For instance, in the case of the hydrogen chloride (HCl) molecule, the chlorine atom is more electronegative than the hydrogen atom. This difference in electronegativity results in a polar covalent bond, with the chlorine atom having a partial negative charge and the hydrogen atom having a partial positive charge. The bond order of the HCl molecule is calculated to be 1.15, reflecting the partial ionic character introduced by the bond polarity.
Comprehending the connection between bond polarity and bond order empowers chemists to accurately predict molecular properties, such as dipole moments and reactivity. This understanding finds practical applications in diverse fields, including materials science, catalysis, and drug design. By harnessing this knowledge, scientists can design and synthesize materials with tailored properties, optimize catalytic processes, and develop targeted therapies for diseases.
Magnetic properties
Magnetic properties, which arise from the movement and spin of electrons, play a significant role in understanding and calculating bond order easily. The magnetic properties of a molecule are influenced by the number and arrangement of unpaired electrons, which in turn affect the overall bond order.
For instance, in a molecule with unpaired electrons, the magnetic field generated by these electrons can interact with external magnetic fields. This interaction can provide valuable insights into the electronic structure of the molecule, including the bond order. By analyzing the magnetic properties of a molecule, chemists can determine the number of unpaired electrons and infer the bond order accordingly.
Furthermore, magnetic properties can also be used to probe the reactivity and bonding behavior of molecules. Paramagnetic molecules, which have unpaired electrons, tend to be more reactive than diamagnetic molecules, which have all electrons paired. Understanding the magnetic properties of molecules can help chemists predict their reactivity and design materials with specific magnetic properties for applications in catalysis, data storage, and spintronics.
In summary, magnetic properties provide a valuable tool for calculating bond order easily and understanding the electronic structure and behavior of molecules. By harnessing this knowledge, chemists can gain insights into molecular reactivity, design materials with tailored properties, and explore novel applications in various fields.
Reactivity
Reactivity, a fundamental property of molecules, describes their tendency to undergo chemical reactions and form new bonds. Understanding reactivity is crucial in various fields, including chemistry, materials science, and drug design. It provides insights into the behavior of molecules, their stability, and their potential applications.
Reactivity is closely connected to the concept of bond order. Bond order, which represents the number of electron pairs shared between two atoms, directly influences the strength and stability of a chemical bond. In general, molecules with lower bond orders are more reactive because the bonds are weaker and more susceptible to breaking. Conversely, molecules with higher bond orders are less reactive due to the stronger bonds.
For example, consider the reaction between hydrogen and oxygen to form water. Hydrogen and oxygen molecules have low bond orders, making them highly reactive. When these molecules come into contact, their atoms readily rearrange to form water molecules, which have higher bond orders and are more stable. This reaction highlights the relationship between bond order and reactivity.
Understanding the connection between reactivity and bond order has practical applications in various fields. In materials science, it enables the design of materials with tailored reactivities for specific applications, such as catalysts or protective coatings. In drug design, it helps researchers develop drugs that are more stable and less reactive, reducing side effects and improving efficacy. Overall, comprehending this relationship empowers scientists to manipulate and control chemical reactions, leading to advancements in diverse areas of science and technology.
Spectroscopic properties
Spectroscopic properties, which arise from the interaction of electromagnetic radiation with matter, provide valuable insights into the electronic structure and bonding of molecules. These properties are closely connected to bond order, a fundamental concept in chemistry that describes the strength and nature of chemical bonds. Understanding the relationship between spectroscopic properties and bond order is essential for calculating bond order easily and accurately.
Spectroscopic techniques, such as ultraviolet-visible (UV-Vis) spectroscopy, infrared (IR) spectroscopy, and nuclear magnetic resonance (NMR) spectroscopy, allow chemists to probe the electronic transitions, vibrational modes, and nuclear spin states of molecules, respectively. These techniques provide information about the energy levels, bond lengths, and atomic environments within a molecule. By analyzing the spectroscopic data, chemists can infer the bond order and gain insights into the molecular structure and bonding.
For example, in UV-Vis spectroscopy, the absorption of light at specific wavelengths corresponds to the electronic transitions between different energy levels in a molecule. The energy difference between these levels is related to the bond order, with higher bond orders typically resulting in higher energy transitions. Similarly, in IR spectroscopy, the vibrational frequencies of bonds can be correlated to bond order, with stronger bonds exhibiting higher vibrational frequencies. These relationships allow chemists to use spectroscopic data to calculate bond order easily and non-invasively.
Understanding the connection between spectroscopic properties and bond order has practical applications in various fields. In inorganic chemistry, it enables the identification and characterization of coordination complexes and metal-organic frameworks. In organic chemistry, it helps elucidate the structure and bonding of complex organic molecules, such as polymers and natural products. Furthermore, in biochemistry, spectroscopic techniques are used to study the structure and dynamics of proteins, DNA, and other biological molecules.
Thermodynamic properties
Thermodynamic properties are a crucial aspect of understanding and calculating bond order easily. They provide insights into the energy changes associated with bond formation and breaking, enabling chemists to predict the stability and reactivity of molecules.
- Enthalpy
Enthalpy, denoted by H, represents the total thermal energy of a system. It encompasses the internal energy and the work done by the system. In the context of bond order, enthalpy changes associated with bond formation or breaking can provide valuable information about bond strength and stability. - Entropy
Entropy, denoted by S, measures the degree of disorder or randomness in a system. Higher entropy corresponds to a more disordered state. Bond formation typically leads to a decrease in entropy, as the atoms become more organized and restricted in their movement. - Gibbs free energy
Gibbs free energy, denoted by G, combines enthalpy and entropy to predict the spontaneity and equilibrium of a reaction. A negative change in Gibbs free energy indicates a spontaneous reaction and a tendency towards bond formation. - Heat capacity
Heat capacity, denoted by C, measures the amount of heat required to raise the temperature of a system by one unit. Bond order influences heat capacity, as stronger bonds typically require more energy to break and result in a lower heat capacity.
Understanding the relationship between thermodynamic properties and bond order empowers chemists to calculate bond order accurately and predict the behavior of molecules. By considering the energy changes, entropy changes, and spontaneity of bond formation, chemists can gain valuable insights into molecular stability, reactivity, and potential applications.
Frequently Asked Questions on Calculating Bond Order Easily
This section addresses common queries and clarifies important aspects of calculating bond order easily.
Question 1: What is the significance of atomic orbital overlap in bond order calculations?
Answer: Atomic orbital overlap determines the extent of electron sharing between atoms, which directly influences bond order. Greater overlap leads to stronger bonds and higher bond orders.
Question 2: How does electron configuration affect bond order?
Answer: Electron configuration governs the number and type of bonds an atom can form. By understanding the electron configuration, chemists can predict the maximum bond order possible.
Question 3: What is the relationship between molecular symmetry and bond order?
Answer: Molecular symmetry dictates the arrangement of orbitals and the resulting bond order. Symmetrical molecules exhibit predictable bond orders based on the symmetry of their atomic orbitals.
Question 4: How is bond length connected to bond order?
Answer: Bond length and bond order are inversely related. Shorter bond lengths indicate greater orbital overlap and, consequently, higher bond orders.
Question 5: Why is bond polarity important in bond order calculations?
Answer: Bond polarity arises from unequal electron sharing, which affects the bond order. Considering bond polarity ensures accurate bond order calculations.
Question 6: How can magnetic properties provide insights into bond order?
Answer: Magnetic properties stem from the movement and spin of electrons. Analyzing these properties can reveal the number of unpaired electrons and infer the bond order accordingly.
These FAQs offer a concise overview of key concepts related to calculating bond order easily. Delving deeper into these topics will further enhance your understanding and enable you to accurately determine bond orders in diverse chemical systems.
In the next section, we will explore advanced techniques for calculating bond order, including resonance and molecular orbital theory.
Tips for Calculating Bond Order Easily
This section provides practical tips to assist you in calculating bond order accurately and efficiently:
Tip 1: Understand Atomic Orbital Overlap
Recognize that the extent of atomic orbital overlap directly influences bond order. Greater overlap leads to stronger bonds and higher bond orders.
Tip 2: Consider Electron Configuration
Electron configuration determines the number and type of bonds an atom can form. By understanding the electron configuration, you can predict the maximum bond order possible.
Tip 3: Analyze Molecular Symmetry
Molecular symmetry dictates the arrangement of orbitals and the resulting bond order. Symmetrical molecules exhibit predictable bond orders based on the symmetry of their atomic orbitals.
Tip 4: Evaluate Bond Length
Bond length is inversely related to bond order. Shorter bond lengths indicate greater orbital overlap and, consequently, higher bond orders.
Tip 5: Account for Bond Polarity
Bond polarity arises from unequal electron sharing, which affects the bond order. Considering bond polarity ensures accurate bond order calculations.
These tips empower you to calculate bond order easily and accurately. Understanding these concepts will enhance your ability to predict molecular properties and chemical reactivity.
In the following section, we will delve into advanced techniques for calculating bond order, including resonance and molecular orbital theory, to further your understanding and problem-solving capabilities.
Conclusion
Throughout this article, we have explored various aspects of calculating bond order easily, providing insights into key concepts and their interconnections. By understanding atomic orbital overlap, electron configuration, molecular symmetry, bond length, and bond polarity, we are well-equipped to accurately determine bond orders in diverse chemical systems.
In summary, the ability to calculate bond order easily empowers chemists to predict molecular properties, understand chemical reactivity, and design new materials with tailored properties. This knowledge finds practical applications in fields such as catalysis, drug design, and materials science, contributing to advancements in science and technology.
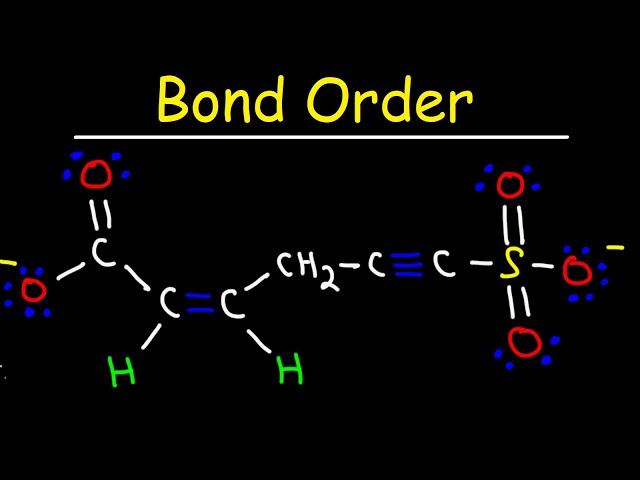