Bond order calculation is a fundamental skill in understanding the nature of chemical bonds. It allows chemists to determine the strength and stability of bonds between atoms, which is crucial for predicting the properties and reactivity of molecules.
This technique is particularly useful in the field of organic chemistry, where it helps chemists comprehend the electronic structure and behavior of organic compounds. A key historical development in this area was the establishment of the Lewis structure method, which enabled chemists to visualize and understand the distribution of electrons in molecules.
In this article, we will delve into the details of how to calculate bond order from Lewis structure. We will explore the step-by-step process, discuss the significance of bond order, and provide practical examples to solidify your understanding.
How to Calculate Bond Order from Lewis Structure
Understanding how to calculate bond order from Lewis structure is essential for chemists to determine the strength and stability of chemical bonds. Key aspects include:
- Electron distribution
- Number of shared electrons
- Type of Lewis structure
- Resonance
- Hybridization
- Bond length
- Bond energy
- Molecular geometry
These aspects provide a comprehensive framework for calculating bond order, which is crucial for predicting the properties and reactivity of molecules. By considering the electron distribution and shared electrons in a Lewis structure, chemists can determine the bond order and gain insights into the nature of chemical bonds.
Electron Distribution
Electron distribution plays a pivotal role in calculating bond order from Lewis structures. It refers to the arrangement and behavior of electrons within a molecule, which determines the strength and nature of chemical bonds.
- Valence Electrons: These are the electrons in the outermost shell of an atom, which participate in chemical bonding. They are crucial for determining the bonding capacity and reactivity of an atom.
- Lone Pairs: These are pairs of electrons that are not involved in covalent bonding. They can influence the geometry and polarity of a molecule.
- Bonding Electrons: These are the electrons that are shared between atoms, forming covalent bonds. The number of bonding electrons determines the bond order.
- Resonance: This occurs when a molecule has multiple Lewis structures with different electron distributions. Resonance can affect bond order and molecular properties.
Understanding electron distribution is essential for accurately calculating bond order and comprehending the electronic structure and bonding behavior of molecules. By considering the distribution and interactions of electrons, chemists can gain valuable insights into the nature of chemical bonds and predict the properties and reactivity of compounds.
Number of shared electrons
In the realm of Lewis structures and chemical bonding, the number of shared electrons plays a pivotal role in determining the bond order. Bond order is a fundamental concept that reflects the strength and stability of a chemical bond, and it is directly influenced by the number of shared electrons between the bonded atoms.
The relationship between the number of shared electrons and bond order is straightforward: the more shared electrons, the higher the bond order. This is because shared electrons create an electrostatic attraction between the positively charged nuclei of the bonded atoms, drawing them closer together and strengthening the bond. Consequently, a double bond, with two shared electrons, is stronger than a single bond, with only one shared electron. Likewise, a triple bond, with three shared electrons, is even stronger than a double bond.
Real-life examples of the connection between the number of shared electrons and bond order abound in the world of chemistry. For instance, the diatomic molecule of hydrogen (H2) has a single bond between the two hydrogen atoms, resulting from the sharing of two electrons. In contrast, the carbon-carbon bond in ethene (C2H4) is a double bond due to the sharing of four electrons between the two carbon atoms. Moreover, the nitrogen-nitrogen bond in dinitrogen (N2) is a triple bond, reflecting the sharing of six electrons between the two nitrogen atoms.
Understanding the relationship between the number of shared electrons and bond order is essential for chemists to predict the properties and reactivity of molecules. By accurately determining the bond order, chemists can gain insights into the strength and stability of chemical bonds, which can inform a wide range of applications, including the design of new materials, the development of drugs, and the understanding of biological processes.
Type of Lewis structure
The type of Lewis structure, which depicts the bonding and arrangement of atoms and electrons within a molecule, plays a critical role in calculating bond order from Lewis structures. Lewis structures can vary depending on factors such as the number of valence electrons, the presence of lone pairs, and the hybridization of atomic orbitals.
The type of Lewis structure directly influences the number of shared electrons between bonded atoms, which in turn determines the bond order. For instance, a single bond, denoted as a single line in a Lewis structure, represents the sharing of two electrons between two atoms, resulting in a bond order of one. In contrast, a double bond, represented by two lines, indicates the sharing of four electrons and a bond order of two. Similarly, a triple bond, depicted by three lines, corresponds to the sharing of six electrons and a bond order of three.
Real-life examples illustrate the connection between Lewis structure type and bond order calculation. Consider the Lewis structures of ethene (C2H4) and benzene (C6H6). Ethene has a double bond between the two carbon atoms, as depicted by two lines in its Lewis structure, indicating a bond order of two. In contrast, benzene has alternating single and double bonds between the carbon atoms, resulting in a resonance hybrid structure with an average bond order of 1.5. These examples demonstrate how the type of Lewis structure provides essential information for calculating bond order and understanding the nature of chemical bonds.
Resonance
Resonance is a crucial concept in chemistry that plays a significant role in calculating bond order from Lewis structures. It arises when a molecule or ion has multiple valid Lewis structures, which means that the electrons are delocalized and cannot be assigned to a specific atom or bond.
- Equivalent Lewis Structures: Resonance structures are different Lewis structures of the same molecule or ion that have the same number of valence electrons. These structures differ only in the placement of double bonds and lone pairs.
- Delocalized Electrons: Resonance involves the delocalization of electrons, which means that the electrons are not confined to a specific bond or atom but are spread out over several atoms or bonds.
- Average Bond Order: For resonance structures, the actual bond order is an average of the bond orders in the individual resonance structures. This average bond order provides a more accurate representation of the electron distribution and bonding in the molecule.
- Benzene Example: A classic example of resonance is benzene, which has six equivalent resonance structures. The electrons in the benzene ring are delocalized over the six carbon atoms, resulting in an average bond order of 1.5 for each carbon-carbon bond.
Resonance provides a deeper understanding of the electronic structure and bonding in molecules and ions. By considering resonance structures, chemists can more accurately calculate bond orders and predict the properties and reactivity of compounds.
Hybridization
Hybridization, a fundamental concept in chemistry, plays a crucial role in understanding how to calculate bond order from Lewis structures. It involves the mixing of atomic orbitals to form new hybrid orbitals with different shapes and energies, which ultimately influences the bonding behavior and properties of molecules.
- Types of Hybridization: The type of hybridization depends on the number and type of atomic orbitals involved in the hybridization process. Common types include sp3, sp2, and sp hybridization.
- Molecular Geometry: Hybridization determines the molecular geometry and shape of a molecule. For instance, sp3 hybridization results in a tetrahedral geometry, while sp2 hybridization leads to a trigonal planar geometry.
- Bond Angles: The bond angles between atoms are influenced by the hybridization of the atomic orbitals involved. Hybridization can alter the bond angles, affecting the overall molecular structure and properties.
- Bond Strength: The strength of a bond is related to the type of hybridization. Hybrid orbitals with more s-character generally form stronger bonds due to better overlap.
Understanding hybridization provides valuable insights into the electronic structure, bonding, and properties of molecules. By considering the hybridization of atomic orbitals, chemists can accurately calculate bond order from Lewis structures, predict molecular geometry, and explain various chemical phenomena.
Bond length
Bond length, a crucial aspect in calculating bond order from Lewis structures, refers to the distance between the nuclei of two bonded atoms. Understanding bond length is vital for comprehending the nature and strength of chemical bonds.
- Atomic Radii: The radii of individual atoms influence bond length. Generally, larger atoms lead to longer bond lengths.
- Bond Order: Bond order and bond length are inversely related. Higher bond orders correspond to shorter bond lengths due to increased electron density and stronger attraction between nuclei.
- Hybridization: The hybridization of atomic orbitals affects bond length. Hybrid orbitals with more s-character result in shorter bond lengths.
- Electronegativity: The electronegativity of bonded atoms can influence bond length. Bonds between atoms with significant electronegativity differences tend to be shorter.
Understanding the relationship between bond length and the aforementioned factors is crucial for accurate bond order calculation. By considering these aspects, chemists can gain valuable insights into the electronic structure and bonding behavior of molecules, enabling them to predict properties and reactivity.
Bond energy
Bond energy plays a fundamental role in understanding how to calculate bond order from Lewis structures. Bond order, a measure of the strength and stability of a chemical bond, is directly related to bond energy. Higher bond orders correspond to stronger bonds, which in turn have higher bond energies.
The bond energy of a molecule is the amount of energy required to break the bond between two atoms. It is typically expressed in kilojoules per mole (kJ/mol). Factors that influence bond energy include the type of bond (single, double, or triple), the electronegativity of the bonded atoms, and the hybridization of the atomic orbitals involved in the bond.
Understanding the relationship between bond energy and bond order is essential for predicting the stability and reactivity of molecules. For example, molecules with higher bond orders are generally more stable and less reactive because more energy is required to break their bonds. Conversely, molecules with lower bond orders are less stable and more reactive. This knowledge is valuable in various fields, including chemistry, materials science, and biochemistry.
Molecular geometry
Molecular geometry plays a crucial role in determining the bond order of a molecule, which provides insights into the strength and stability of chemical bonds. The arrangement of atoms in space, known as molecular geometry, influences the overlap of atomic orbitals and the distribution of electrons, which ultimately affects the bond order.
For example, in a linear molecule like carbon dioxide (CO2), the two oxygen atoms are bonded to the central carbon atom in a linear fashion. This geometry results in sp hybridization of the carbon atom, leading to a double bond between the carbon and each oxygen atom. The double bond has a bond order of 2, indicating a strong and stable bond.
In contrast, a molecule with a tetrahedral geometry, such as methane (CH4), has four hydrogen atoms bonded to the central carbon atom in a tetrahedral arrangement. The carbon atom undergoes sp3 hybridization, forming four single bonds with the hydrogen atoms. Each single bond has a bond order of 1, representing a weaker bond compared to the double bond in CO2.
Understanding the relationship between molecular geometry and bond order is essential for predicting the properties and reactivity of molecules. It enables chemists to design and synthesize molecules with specific properties for various applications, such as pharmaceuticals, materials science, and energy storage.
Frequently Asked Questions
This section addresses common questions and clarifies aspects related to calculating bond order from Lewis structures.
Question 1: What is bond order, and why is it important?
Bond order is a measure of the strength and stability of a chemical bond. It indicates the number of electron pairs shared between two atoms, providing insights into the properties and reactivity of molecules.
Question 2: How do I determine the bond order between two atoms from a Lewis structure?
Count the number of lines connecting the two atoms. A single line represents a bond order of 1, a double line represents a bond order of 2, and so on.
Question 3: Can resonance affect bond order?
Yes. Resonance occurs when a molecule has multiple valid Lewis structures. The bond order in resonance structures is an average of the bond orders in the individual structures.
Question 4: How does hybridization influence bond order?
Hybridization involves mixing atomic orbitals to form new hybrid orbitals. Different types of hybridization (e.g., sp3, sp2) affect the overlap of orbitals and consequently the bond order.
Question 5: What is the relationship between bond order and bond length?
Bond order is inversely proportional to bond length. Higher bond orders generally correspond to shorter bond lengths due to increased electron density and stronger attraction between nuclei.
Question 6: How can I use bond order to predict molecular properties?
Bond order provides insights into molecular stability, reactivity, and other properties. Molecules with higher bond orders tend to be more stable and less reactive.
These FAQs provide a foundation for understanding how to calculate bond order from Lewis structures. Further exploration of advanced concepts and applications is recommended for a comprehensive grasp of the topic.
Continuing to the next section, we will delve into advanced aspects of bond order calculations, including resonance structures, hybridization effects, and applications in predicting molecular properties.
Tips for Calculating Bond Order from Lewis Structures
This section provides practical tips to enhance your understanding and accuracy in calculating bond order from Lewis structures.
Tip 1: Familiarize yourself with the concept of bond order and its significance.
Tip 2: Practice identifying single, double, and triple bonds in Lewis structures.
Tip 3: Pay attention to resonance structures and calculate the average bond order when applicable.
Tip 4: Consider the hybridization of atoms to determine the type and strength of bonds.
Tip 5: Utilize reference materials or online tools for quick calculations.
Tip 6: Relate bond order to bond length, bond energy, and molecular geometry.
Tip 7: Apply your knowledge to predict the stability and reactivity of molecules.
Tip 8: Seek guidance from experienced professionals or consult reliable sources for further clarification.
These tips will equip you with a solid foundation for calculating bond order from Lewis structures and enable you to explore more advanced concepts in chemical bonding.
In the concluding section of this article, we will delve into applications of bond order calculations, showcasing its practical relevance in various scientific fields.
Conclusion
In summary, calculating bond order from Lewis structures provides valuable insights into the nature of chemical bonds. By understanding the number of shared electrons, type of Lewis structure, resonance, hybridization, and other factors, chemists can accurately determine bond order and gain insights into molecular properties such as stability, reactivity, and geometry.
This comprehensive understanding of bond order enables chemists to design and synthesize molecules with specific properties for diverse applications in fields such as materials science, pharmaceuticals, and energy storage. Additionally, bond order calculations contribute to a deeper comprehension of chemical reactions and processes, aiding in the advancement of scientific research and technological innovations.
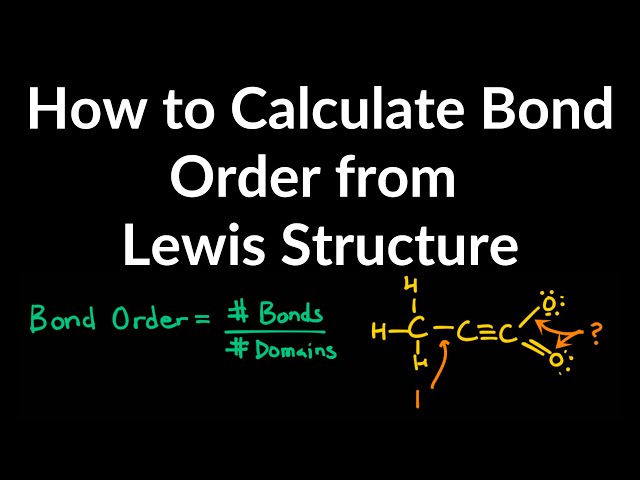