How to calculate bond order in CO2 is a crucial aspect of understanding the molecular structure and bonding characteristics of this important molecule.
Bond order is a measure of the strength of a chemical bond, and understanding how to calculate it can provide insights into the reactivity and stability of molecules. In this context, CO2 serves as a significant example, as its bond order has implications for its role in atmospheric chemistry, carbon sequestration, and plant photosynthesis.
Throughout history, the development of methods for calculating bond order has played a pivotal role in advancing our understanding of molecular behavior and chemical reactivity.
How to Calculate Bond Order in CO2
Understanding how to calculate bond order in CO2 is essential for comprehending its molecular structure and bonding characteristics.
- Chemical Bonding
- Molecular Orbitals
- Valence Electrons
- Hybridization
- Resonance
- Lewis Structures
- Quantum Mechanics
- Computational Chemistry
- Spectroscopy
- Thermodynamics
These aspects provide a comprehensive framework for calculating bond order in CO2, encompassing fundamental concepts in chemistry and leveraging advanced computational techniques. By exploring these aspects in detail, we gain deeper insights into the nature of chemical bonding and the behavior of molecules like CO2.
Chemical Bonding
Chemical bonding is the process of atoms interacting with each other to form molecules. It is the “glue” that holds molecules together and determines their structure and properties. To accurately calculate bond order in CO2, it is essential to have a firm understanding of the principles of chemical bonding, as they govern the number and strength of bonds between atoms.
- Types of Bonds
There are different types of chemical bonds, including covalent bonds, ionic bonds, and metallic bonds. Covalent bonds involve the sharing of electrons between atoms, while ionic bonds involve the transfer of electrons from one atom to another. Metallic bonds are formed by the attraction between positively charged metal ions and mobile electrons. - Bond Strength
The strength of a chemical bond is determined by the number of electrons shared between atoms and the electronegativity of the atoms involved. Electronegativity is a measure of an atom’s ability to attract electrons, and the greater the difference in electronegativity between two atoms, the stronger the bond between them. - Bond Length
The length of a chemical bond is the distance between the nuclei of the bonded atoms. Bond length is inversely related to bond strength, meaning that shorter bonds are stronger than longer bonds. - Bond Order
Bond order is a measure of the number of chemical bonds between two atoms. It can be calculated using a variety of methods, including the molecular orbital theory and the valence bond theory.
By understanding the principles of chemical bonding, we can gain insights into the structure and properties of molecules like CO2. This knowledge is essential for predicting the reactivity and behavior of molecules in various chemical systems and processes.
Molecular Orbitals
Molecular orbitals play a crucial role in calculating bond order in CO2, providing a theoretical framework for understanding the electronic structure and bonding interactions within the molecule.
- Atomic Orbitals: The foundation of molecular orbitals, atomic orbitals describe the wave-like behavior of electrons around individual atoms.
- Linear Combination of Atomic Orbitals (LCAO): Molecular orbitals are formed by combining atomic orbitals, resulting in new orbitals that extend over the entire molecule.
- Bonding and Antibonding Orbitals: Molecular orbitals can be classified as bonding orbitals (promote bonding) or antibonding orbitals (weaken bonding) based on their symmetry and electron density.
- Molecular Orbital Energy Levels: The energy levels of molecular orbitals determine the stability and reactivity of the molecule.
By considering the molecular orbitals of CO2, we can determine the number and type of bonds between the carbon and oxygen atoms, providing a quantitative measure of bond order. This understanding is essential for predicting the properties and behavior of CO2 in various chemical and biological systems.
Valence Electrons
Valence electrons are the electrons in an atom’s outermost shell, and they play a critical role in determining the chemical properties of an element. In the context of calculating bond order in CO2, valence electrons are essential for understanding the formation and strength of the chemical bonds between the carbon and oxygen atoms.
The number of valence electrons an atom has determines its bonding capacity. For example, carbon has four valence electrons, meaning it can form four covalent bonds with other atoms. Oxygen has six valence electrons, allowing it to form two covalent bonds. In the case of CO2, the carbon atom shares two of its valence electrons with each oxygen atom, resulting in the formation of two double bonds between the carbon and oxygen atoms.
Understanding the role of valence electrons is crucial for accurately calculating bond order in CO2. Bond order is a measure of the strength of a chemical bond, and it is determined by the number of electron pairs shared between the bonded atoms. In the case of CO2, each double bond consists of two shared electron pairs, giving a bond order of two for each carbon-oxygen bond.
The concept of valence electrons and bond order has practical applications in various fields, including chemistry, materials science, and biochemistry. By understanding the valence electron configurations of atoms, scientists can predict the chemical reactivity of molecules and design new materials with specific properties.
Hybridization
Hybridization is the process of combining atomic orbitals to form new hybrid orbitals with different shapes and energies. This concept plays a critical role in understanding how to calculate bond order in CO2 because it determines the geometry and bonding characteristics of the molecule.
In the case of CO2, the carbon atom undergoes sp hybridization, which involves the mixing of one 2s orbital and two 2p orbitals to form three equivalent sp hybrid orbitals. These hybrid orbitals then overlap with the p orbitals of the two oxygen atoms to form two double bonds, resulting in a linear molecular geometry.
Understanding hybridization is essential for accurately calculating bond order in CO2. Bond order is a measure of the strength and number of bonds between atoms, and it is determined by the number of electron pairs shared between them. In CO2, each carbon-oxygen double bond consists of two shared electron pairs, giving a bond order of two.
The concept of hybridization has broad applications in chemistry, including predicting molecular geometry, understanding bonding in complex molecules, and designing new materials. By understanding hybridization, scientists can gain insights into the structure and properties of molecules, which is crucial for advancing fields such as drug design, materials science, and catalysis.
Resonance
Resonance is a crucial concept in understanding how to calculate bond order in CO2 as it provides a means to describe the delocalization of electrons within the molecule.
- Equivalent Lewis Structures
Resonance structures are alternative Lewis structures that represent the same molecule, differing only in the placement of electrons. In the case of CO2, there are two resonance structures that contribute to the overall electronic structure.
- Delocalized Electrons
Resonance involves the delocalization of electrons, meaning that the electrons are not confined to a specific bond or atom. This delocalization results in a more stable and lower-energy molecule.
- Bond Order
Resonance affects the bond order in CO2. The bond order is a measure of the strength and number of bonds between atoms, and in the case of CO2, resonance results in a bond order of 1.5 for each carbon-oxygen bond.
- Molecular Properties
Resonance can influence various molecular properties, such as bond lengths, vibrational frequencies, and reactivity. Understanding resonance is essential for accurately predicting and interpreting these properties.
By considering resonance, we gain a more complete picture of the electronic structure of CO2, which is crucial for understanding its chemical bonding and behavior. Resonance is a fundamental concept in chemistry, and its applications extend far beyond CO2, playing a vital role in describing the structure and properties of many other molecules.
Lewis Structures
Lewis structures are an essential tool for understanding how to calculate bond order in CO2. They provide a visual representation of the distribution of electrons in a molecule, which is crucial for determining the number and strength of bonds between atoms. In the case of CO2, the Lewis structure shows that the carbon atom is bonded to two oxygen atoms by double bonds. Each double bond consists of two shared electron pairs, resulting in a bond order of two for each carbon-oxygen bond.
Without understanding Lewis structures, it would be difficult to determine the bond order in CO2. The Lewis structure provides a clear picture of the electron distribution and bonding arrangement within the molecule, allowing us to count the number of shared electron pairs and calculate the bond order accordingly.
Lewis structures also have practical applications in various fields, including chemistry, materials science, and biochemistry. By understanding Lewis structures, scientists can predict the reactivity of molecules, design new materials with specific properties, and understand the mechanisms of biological processes. The connection between Lewis structures and bond order calculation is a fundamental aspect of chemistry, providing a powerful tool for understanding and predicting molecular behavior.
Quantum Mechanics
Understanding quantum mechanics is crucial for accurately calculating bond order in CO2 as it provides the theoretical framework for describing the behavior of electrons within molecules.
- Wave-Particle Duality
Electrons exhibit both wave-like and particle-like properties, and quantum mechanics provides a mathematical framework to describe this dual nature and its implications for bonding in CO2.
- Electron Configuration
Quantum mechanics helps determine the electron configuration of atoms, including the number and arrangement of electrons in atomic orbitals. This information is essential for understanding the bonding interactions in CO2 and calculating bond order.
- Molecular Orbitals
Quantum mechanics provides the concept of molecular orbitals, which describe the distribution of electrons within a molecule and their contribution to bonding. By understanding the molecular orbitals of CO2, we can determine the bond order and predict its molecular properties.
- Quantum Superposition
Quantum mechanics introduces the concept of quantum superposition, where electrons can exist in multiple states or locations simultaneously. This concept has implications for understanding the delocalized nature of electrons in CO2 and the resulting bond order.
Quantum mechanics provides a powerful lens through which we can understand the electronic structure and bonding in CO2. By incorporating its principles into our calculations, we gain a more accurate and comprehensive picture of bond order and its implications for the molecule’s properties and behavior.
Computational Chemistry
Computational chemistry plays a pivotal role in the accurate calculation of bond order in CO2. It provides powerful computational tools and methodologies that enable us to model and simulate the electronic structure and bonding interactions within the CO2 molecule. By leveraging the principles of quantum mechanics, computational chemistry allows us to solve complex mathematical equations that describe the behavior of electrons and their interactions with atomic nuclei.
One of the key methods used in computational chemistry for bond order calculations is density functional theory (DFT). DFT is a powerful tool that combines quantum mechanics with classical physics to approximate the electron density distribution within a molecule. This electron density distribution provides valuable insights into the bonding characteristics of the molecule, including the bond order. By employing sophisticated algorithms and high-performance computing resources, DFT calculations can accurately predict the bond order in CO2, taking into account the effects of electron correlation and other factors that influence bonding.
Computational chemistry has proven invaluable in advancing our understanding of bond order in CO2 and other molecules. It has enabled scientists to investigate the effects of various factors, such as molecular geometry, substituents, and external fields, on bond order. This understanding has led to the development of new materials with tailored properties and the optimization of chemical processes involving CO2. Moreover, computational chemistry has provided a deeper understanding of the electronic structure and bonding in CO2, contributing to the broader field of chemistry and our knowledge of molecular interactions.
Spectroscopy
Spectroscopy is a powerful technique used to analyze the interaction of light with matter, providing valuable insights into the electronic structure, vibrational modes, and chemical composition of molecules. Spectroscopy plays a critical role in helping us understand and calculate bond order in CO2.
One of the most common spectroscopic techniques used for bond order determination is infrared (IR) spectroscopy. IR spectroscopy measures the absorption of infrared radiation by molecules, which corresponds to the excitation of vibrational modes. The frequency of the absorbed radiation is directly related to the strength of the chemical bond, and thus provides indirect information about bond order. By analyzing the IR spectrum of CO2, we can identify the vibrational modes associated with the C=O bond and determine its bond order.
Another important spectroscopic technique is Raman spectroscopy. Raman spectroscopy measures the inelastic scattering of light by molecules, which provides information about molecular vibrations and other low-frequency excitations. Similar to IR spectroscopy, Raman spectroscopy can be used to determine the bond order in CO2 by analyzing the vibrational modes of the molecule.
Spectroscopy provides crucial experimental data that complements theoretical calculations and models used to determine bond order in CO2. By combining spectroscopic measurements with computational methods, we can obtain a more comprehensive understanding of the electronic structure and bonding characteristics of CO2. This understanding is essential for predicting the reactivity and properties of CO2, which has implications in fields such as atmospheric chemistry, carbon capture, and materials science.
Thermodynamics
Thermodynamics, a branch of physics concerned with heat and its relation to other forms of energy, plays a crucial role in understanding how to calculate bond order in CO2. Thermodynamics provides a framework for understanding the energetics of chemical reactions, including the formation and breaking of chemical bonds.
In the context of CO2, thermodynamics helps us determine the stability and reactivity of the molecule. By analyzing the enthalpy and entropy changes associated with the formation of CO2, we can gain insights into the bond order and the strength of the carbon-oxygen bonds. This information is essential for understanding the behavior of CO2 in various chemical and biological processes.
For example, in combustion reactions, the thermodynamics of CO2 formation is critical for determining the efficiency and heat output of the process. By understanding the bond order and stability of CO2, engineers can optimize combustion systems to maximize energy production and minimize harmful emissions. Additionally, in carbon capture and storage technologies, thermodynamics plays a vital role in understanding the stability of CO2 under different storage conditions, ensuring the effectiveness and safety of these technologies.
Overall, thermodynamics provides a powerful tool for understanding and calculating bond order in CO2. By considering the energetics and equilibrium of chemical reactions, we can gain valuable insights into the molecular structure, stability, and reactivity of CO2, leading to advancements in various fields such as energy, environmental science, and materials chemistry.
Frequently Asked Questions about Calculating Bond Order in CO2
This FAQ section addresses common questions and provides clear explanations to enhance your understanding of how to calculate bond order in CO2.
Question 1: What is bond order and why is it important in CO2?
Answer: Bond order describes the strength and multiplicity of chemical bonds between atoms. In CO2, bond order helps us understand the stability and reactivity of the molecule, which is crucial in fields like atmospheric chemistry and carbon capture.
Question 2: What methods can be used to calculate bond order in CO2?
Answer: Various methods exist, including the valence bond theory, molecular orbital theory, and density functional theory (DFT). Each method provides a unique approach to calculating bond order based on different theoretical frameworks.
Question 3: How does hybridization affect bond order in CO2?
Answer: Hybridization involves the mixing of atomic orbitals to form new hybrid orbitals. In CO2, the carbon atom undergoes sp hybridization, which influences the geometry and bond order of the molecule.
Question 4: What role does resonance play in calculating bond order in CO2?
Answer: Resonance involves the delocalization of electrons across multiple Lewis structures. In CO2, resonance results in a fractional bond order, indicating the resonance hybrid’s stability and lower energy compared to a single Lewis structure.
Question 5: How can experimental techniques contribute to understanding bond order in CO2?
Answer: Spectroscopic techniques like IR and Raman spectroscopy provide valuable insights into bond order by analyzing the vibrational modes and energy levels of CO2. These experimental data complement theoretical calculations and enhance our understanding of the molecule’s bonding characteristics.
Question 6: Why is calculating bond order in CO2 relevant in practical applications?
Answer: Understanding bond order in CO2 has implications for diverse fields. In atmospheric chemistry, it helps predict CO2‘s behavior and its role in climate change. In materials science, it aids in designing new materials with tailored properties, and in carbon capture technologies, it informs strategies for efficient CO2 storage.
These FAQs provide a deeper understanding of how to calculate bond order in CO2 and its significance in various scientific disciplines. In the next section, we will explore advanced concepts and applications related to bond order calculations.
Tips for Calculating Bond Order in CO2
To ensure accurate and efficient calculation of bond order in CO2, consider the following practical tips:
Tip 1: Understand the theoretical concepts
Grasp the fundamentals of chemical bonding, molecular orbital theory, and hybridization to lay a strong foundation for bond order calculations.
Tip 2: Utilize computational tools
Employ computational chemistry software and databases to leverage advanced algorithms and high-performance computing for precise bond order determination.
Tip 3: Consider resonance and delocalization
In cases of resonance, account for the delocalization of electrons to obtain a more accurate representation of bond order and molecular stability.
Tip 4: Analyze experimental data
Incorporate experimental data from spectroscopic techniques like IR and Raman spectroscopy to validate theoretical calculations and gain insights into vibrational modes and energy level transitions.
Tip 5: Study chemical reactions and energetics
Examine chemical reactions involving CO2 and analyze the energetics of bond formation and breaking to understand how these factors influence bond order.
By following these tips, you can enhance the accuracy and reliability of your bond order calculations in CO2, leading to a deeper understanding of its molecular structure and chemical behavior.
These insights lay the groundwork for exploring advanced applications of bond order calculations in fields such as atmospheric chemistry, materials science, and carbon capture technologies, as discussed in the subsequent section.
Conclusion
Understanding how to calculate bond order in CO2 provides valuable insights into the molecular structure and chemical bonding of this important molecule. By employing various theoretical and experimental approaches, we gain a deeper comprehension of the electronic structure, hybridization, and resonance effects that shape the CO2 molecule.
Key considerations in bond order calculations include the selection of appropriate computational methods, analysis of experimental data, and understanding the impact of resonance and delocalization. These factors collectively contribute to accurate and reliable determination of bond order, enabling researchers to predict and interpret the behavior of CO2 in diverse chemical systems.
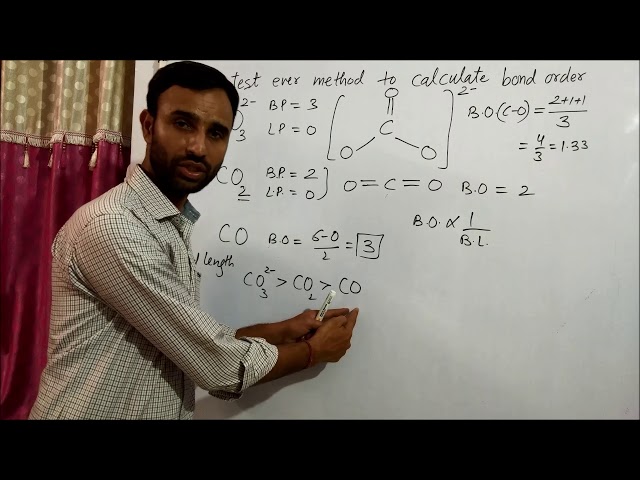