Calculating bond order is essential for understanding molecular structure and chemical bonding. It measures the strength and type of bond between atoms within a molecule and can be used to predict its properties and reactivity. One example is the double bond in ethene (C2H4), which is stronger and shorter than the single bond in ethane (C2H6) due to a higher bond order.
Bond order has significant implications in fields such as materials science, catalysis, and pharmaceutical design. The concept originated in the early 20th century with Lewis’s theory of electron pairs and Langmuir’s theory of the octet rule. Linus Pauling later developed more sophisticated methods to calculate bond order using quantum mechanics.
This article delves into the step-by-step process of calculating bond order, exploring various methods and their applications. It provides a comprehensive understanding of this fundamental concept, empowering readers to analyze and predict the behavior of molecules.
How to Calculate Bond Order of a Molecule
Understanding how to calculate bond order is crucial for chemists to determine the strength and type of chemical bonds between atoms within a molecule. Various aspects play a vital role in these calculations:
- Molecular Orbitals
- Electron Configuration
- Resonance
- Hybridization
- Bond Length
- Bond Energy
- Electronegativity
- Partial Charges
- Quantum Mechanics
These aspects are interconnected and provide insights into the nature of chemical bonding. For instance, the number and type of molecular orbitals available for bonding determine the maximum bond order possible. Resonance structures contribute to bond order by delocalizing electrons and altering bond lengths. Hybridization affects bond order by influencing the overlap of atomic orbitals. By considering these aspects, chemists can accurately calculate bond order, enabling them to predict molecular properties, reactivity, and behavior in chemical reactions.
Molecular Orbitals
Molecular orbitals play a central role in determining the bond order of a molecule. They are mathematical functions that describe the wave-like behavior of electrons within a molecule and provide insights into the electronic structure and bonding.
- Atomic Orbitals: The foundation of molecular orbitals, atomic orbitals describe the wave functions of electrons around individual atoms.
- Linear Combination of Atomic Orbitals (LCAO): Molecular orbitals are formed by combining atomic orbitals, resulting in constructive and destructive interference of electron waves.
- Molecular Orbital Energy Levels: The energy of molecular orbitals depends on the symmetry and overlap of the atomic orbitals that combine to form them.
- Bonding and Antibonding Orbitals: Molecular orbitals can be bonding (lower energy) or antibonding (higher energy), affecting the bond order and stability of the molecule.
Understanding molecular orbitals is crucial for calculating bond order because they determine the number and type of electrons available for bonding. By analyzing the molecular orbital diagram, chemists can predict the bond order, electronic configuration, and properties of a molecule.
Electron Configuration
Electron configuration plays a crucial role in calculating bond order as it determines the number and arrangement of electrons in the molecular orbitals. A molecule’s electron configuration can be represented using various models, including the Lewis structure, molecular orbital diagram, and valence bond theory.
- Atomic Orbitals: Electrons occupy atomic orbitals around the atoms in a molecule. The type and energy of these orbitals influence the formation and properties of molecular orbitals.
- aufbau Principle: Electrons fill atomic orbitals in order of increasing energy, starting with the lowest energy orbitals first. This principle determines the electron configuration of atoms and molecules.
- Hund’s Rule: When multiple electrons occupy degenerate orbitals (orbitals with the same energy), they align their spins parallel to each other. This rule affects the overall spin multiplicity of a molecule.
Understanding electron configuration allows chemists to determine the number of bonding and antibonding electrons in a molecule. This information, combined with the molecular orbital diagram, helps calculate the bond order, predict molecular properties, and explain chemical reactivity.
Resonance
Resonance, a crucial concept in chemistry, profoundly influences how to calculate bond order of a molecule. It arises when a molecule possesses multiple valid Lewis structures, each representing a different distribution of electrons. This phenomenon complicates the traditional view of a molecule with localized bonds and fixed bond orders.
In resonance, the actual electronic structure of the molecule is a hybrid of the contributing Lewis structures. This delocalization of electrons affects the bond lengths and bond orders within the molecule. To accommodate this, resonance structures are weighted according to their relative contributions, and the bond order is calculated as an average over these structures.
For example, in the benzene molecule, resonance occurs due to the delocalization of electrons in the six-membered ring. The Kekule structures, which depict alternating single and double bonds, are two major resonance contributors. However, the actual bond lengths in benzene are all equal, indicating an intermediate bond order between a single and double bond. This is attributed to the resonance hybrid, which distributes the electron density more evenly around the ring.
Understanding resonance is essential for accurately calculating bond orders and predicting molecular properties. It provides insights into the electronic structure and bonding of molecules, which has practical applications in fields such as organic chemistry, materials science, and drug design.
Hybridization
Hybridization is a fundamental concept in chemistry that plays a crucial role in calculating bond order of a molecule. It involves the mixing of atomic orbitals to form new hybrid orbitals with different shapes and energies. This hybridization affects the geometry, bonding, and properties of molecules.
- Types of Hybridization
Common types of hybridization include sp, sp2, sp3, and sp3d. Each type results in a specific geometry and number of hybrid orbitals.
- Molecular Geometry
Hybridization influences the molecular geometry by determining the arrangement of atoms around a central atom. For example, sp3 hybridization leads to a tetrahedral geometry, while sp2 hybridization results in a trigonal planar geometry.
- Bonding
Hybrid orbitals participate in covalent bonding by overlapping with orbitals from other atoms. The number and type of hybrid orbitals available determine the bonding capacity of an atom.
- Bond Order
The extent of orbital overlap in hybrid orbitals affects the bond order. Greater overlap leads to stronger bonds and higher bond order.
Understanding hybridization is essential for accurately calculating bond order and predicting the properties and behavior of molecules. It provides insights into the electronic structure and bonding of molecules, which has applications in various fields of chemistry and materials science.
Bond Length
Bond length is a critical factor in determining the bond order of a molecule. It refers to the distance between the nuclei of two bonded atoms and influences the strength and properties of the bond.
- Bond Type
Bond length varies depending on the type of bond, such as single, double, or triple bonds. Generally, shorter bond lengths indicate stronger bonds and higher bond orders.
- Electronegativity
The electronegativity of the bonded atoms affects bond length. Atoms with higher electronegativity attract electrons more strongly, resulting in shorter bond lengths.
- Hybridization
The hybridization of atomic orbitals influences bond length. Different types of hybridization lead to different orbital overlaps and bond lengths.
- Resonance
Resonance structures can affect bond lengths by delocalizing electrons and altering the bond order. This can lead to shorter or longer bond lengths compared to non-resonant structures.
Understanding bond length is crucial for accurately calculating bond order and predicting the properties of molecules. It provides insights into the electronic structure and bonding of molecules, which has applications in various fields of chemistry and materials science.
Bond Energy
Bond energy is a fundamental property that measures the strength of a chemical bond between two atoms in a molecule. It is the amount of energy required to break a bond and is directly related to the bond order of a molecule.
A higher bond order indicates a stronger bond and a higher bond energy. This is because the bond order represents the number of electron pairs shared between two atoms, and more electron pairs result in a stronger bond. For example, a double bond, with two shared electron pairs, has a higher bond order and bond energy than a single bond, with only one shared electron pair.
Bond energy is a critical component of calculating bond order because it provides a quantitative measure of the bond strength. By knowing the bond energy and the number of bonds between two atoms, one can determine the bond order. This information is essential for understanding the stability and reactivity of molecules.
In practice, bond energy data are used in various fields of chemistry, including thermochemistry, spectroscopy, and molecular modeling. For example, bond energies can be used to predict the products and energy changes of chemical reactions, analyze the vibrational spectra of molecules, and design new materials with desired properties.
Electronegativity
Electronegativity, a crucial property of atoms, plays a significant role in determining the bond order of a molecule. It reflects the ability of an atom to attract electrons towards itself within a chemical bond.
- Electronegativity Scale
The Pauling electronegativity scale quantifies the electronegativity of different elements. It ranges from 0.7 for cesium to 4.0 for fluorine, providing a relative measure of electron-attracting power.
- Bond Polarity
Electronegativity differences between bonded atoms create bond polarity. The more electronegative atom attracts electrons more strongly, resulting in a partial positive charge on the less electronegative atom and a partial negative charge on the more electronegative atom.
- Bond Order
Electronegativity influences bond order by affecting the distribution of electrons in a bond. Higher electronegativity differences lead to more polarized bonds, with electrons shifted towards the more electronegative atom. This can result in a decrease in bond order.
- Resonance
Electronegativity also plays a role in resonance. When electronegative atoms are involved in resonance structures, they tend to stabilize the resonance hybrid by withdrawing electrons. This can affect the overall bond order and the properties of the molecule.
Understanding electronegativity is crucial for accurately calculating bond order and predicting the properties of molecules. By considering the electronegativity of the bonded atoms, chemists can gain insights into bond polarity, electron distribution, and the overall stability of molecules.
Partial Charges
In the context of calculating bond order, partial charges refer to the uneven distribution of electrons within a covalent bond. This concept plays a crucial role in understanding the polarity and properties of molecules.
- Electronegativity Difference
The difference in electronegativity between bonded atoms determines the extent of partial charges. The more electronegative atom attracts electrons more strongly, resulting in a partial negative charge on itself and a partial positive charge on the less electronegative atom.
- Resonance Structures
In resonance structures, electrons are delocalized over multiple atoms. This can lead to the development of partial charges on specific atoms, influencing the overall bond order and molecular properties.
- Polar Functional Groups
The presence of polar functional groups, such as carbonyl groups or hydroxyl groups, can induce partial charges in a molecule. These functional groups create a dipole moment, leading to uneven electron distribution.
- Inductive Effects
Inductive effects occur when electronegative atoms or groups withdraw electrons from adjacent bonds, creating a cascade of partial charges along the molecular framework.
Understanding partial charges is essential for accurately calculating bond order. By considering the factors that influence partial charges, chemists can gain insights into the polarity, electron distribution, and overall stability of molecules.
Quantum Mechanics
Quantum mechanics plays a fundamental role in understanding the electronic structure of molecules and calculating bond orders. It provides a theoretical framework that describes the behavior of electrons at the atomic and molecular level.
- Wave-Particle Duality
Quantum mechanics treats electrons as both particles and waves. The wavefunction of an electron describes its probability of being found in a particular region of space, influencing the formation of molecular orbitals and bond order.
- Molecular Orbitals
Quantum mechanics explains the formation of molecular orbitals by combining atomic orbitals. The energy levels and shapes of these molecular orbitals determine the number and types of bonds formed, directly affecting bond order calculations.
- Electron Spin
Electrons have an intrinsic property called spin, which must be considered when calculating bond order. The Pauli exclusion principle dictates that two electrons in the same orbital must have opposite spins, influencing the electronic configuration and bond formation.
- Quantum Superposition
Quantum mechanics allows electrons to exist in multiple states simultaneously. This concept of superposition affects the delocalization of electrons in resonance structures, influencing bond order calculations by considering the contributions of multiple resonance forms.
Quantum mechanics provides a comprehensive framework for understanding and calculating bond order, enabling chemists to predict and analyze the properties and reactivity of molecules. Its principles guide the development of computational methods and experimental techniques used to probe the electronic structure and bonding in chemical systems.
Frequently Asked Questions about Bond Order Calculation
This section addresses common questions and clarifies key aspects related to calculating bond order in molecules.
Question 1: What is bond order and why is it important?
Answer: Bond order is a measure of the strength and type of bond between atoms in a molecule. It provides insights into molecular structure, stability, and reactivity, guiding chemical analysis and design.
Question 2: How do I calculate bond order using the molecular orbital theory?
Answer: Molecular orbital theory involves analyzing the energy levels and shapes of molecular orbitals to determine the number and type of bonds formed. Bond order is calculated based on the number of electrons occupying bonding and antibonding orbitals.
Question 3: Can resonance affect bond order calculations?
Answer: Yes, resonance occurs when a molecule has multiple valid Lewis structures. Bond order calculations consider the contributions of all resonance structures, providing a more accurate representation of the electronic structure and bond strengths.
Question 4: How does hybridization influence bond order?
Answer: Hybridization involves the mixing of atomic orbitals to form hybrid orbitals with different shapes and energies. The type of hybridization affects the geometry and bonding capacity of atoms, thus influencing the bond order.
Question 5: What role does electronegativity play in bond order calculations?
Answer: Electronegativity measures the ability of atoms to attract electrons in a bond. Differences in electronegativity between bonded atoms create bond polarity and influence the distribution of electrons. This can affect the bond order and the overall polarity of the molecule.
Question 6: How can I use bond order calculations to predict molecular properties?
Answer: Bond order provides valuable information about bond strength, length, and vibrational frequencies. By understanding bond order, chemists can make predictions about molecular stability, reactivity, and behavior in various chemical processes.
These FAQs provide a foundational understanding of bond order calculations, highlighting their significance in chemistry. In the next section, we will explore advanced topics and applications related to bond order, enabling a deeper understanding of molecular structure and bonding.
Tips for Calculating Bond Order
To enhance your understanding and accuracy when calculating bond order, consider these practical tips:
Tip 1: Determine the molecular orbital diagram of the molecule to visualize the energy levels and interactions of molecular orbitals.
Tip 2: Identify the bonding and antibonding orbitals and count the number of electrons occupying each type.
Tip 3: Utilize resonance structures to account for the delocalization of electrons and their impact on bond order.
Tip 4: Consider the hybridization of atomic orbitals, as it affects the geometry and bonding capacity of atoms, influencing bond order.
Tip 5: Understand the electronegativity of bonded atoms, as it affects the distribution of electrons and can alter bond order.
Tip 6: Employ computational methods, such as density functional theory (DFT), to obtain accurate bond orders and insights into the electronic structure of molecules.
Tip 7: Validate your calculated bond orders by comparing them with experimental data or reference values.
By following these tips, you can refine your bond order calculations, leading to a deeper understanding of molecular structure and bonding.
In the next section, we will explore advanced applications of bond order calculations, demonstrating their significance in predicting molecular properties and chemical reactivity.
Conclusion
In summary, understanding how to calculate bond order provides invaluable insights into molecular structure and bonding. By considering molecular orbital theory, resonance, hybridization, electronegativity, and quantum mechanics, chemists can accurately determine bond orders.
Key takeaways include:
Bond order reflects the strength and type of bond between atoms, influencing molecular properties and reactivity.Advanced computational methods and experimental techniques enable precise bond order calculations and analysis of electronic structure.Understanding bond order is essential for predicting molecular behavior, designing new materials, and advancing various fields of chemistry, including organic chemistry, materials science, and medicinal chemistry.Bond order calculations continue to empower researchers and scientists in unraveling the intricacies of molecular bonding and designing novel compounds with tailored properties. As we delve deeper into the quantum realm, further advancements in bond order calculations promise exciting discoveries and transformative applications.
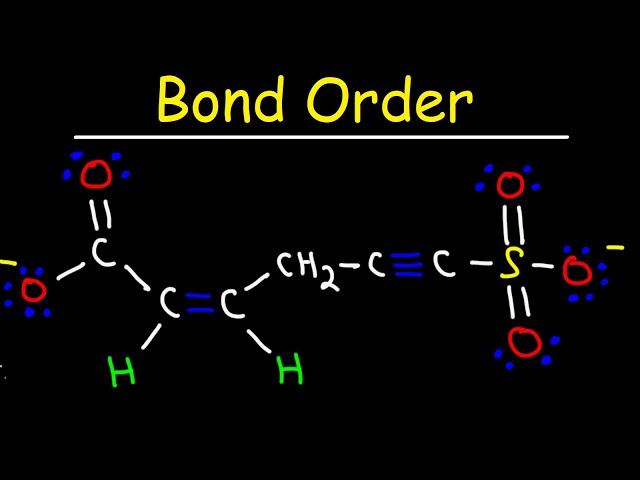