Bond order, a value that describes the strength and stability of chemical bonds, is crucial for understanding molecular properties and reactivity. In this article, we will delve into how to calculate the bond order of the carbonate ion (CO32-), a common and versatile species in various chemical and biological systems.
Determining the bond order of CO32- provides valuable insights into its structure, bonding characteristics, and reactivity. This knowledge is essential in fields such as inorganic chemistry, materials science, and biochemistry, where the behavior of carbonate-containing compounds plays a significant role.
Historically, the concept of bond order was introduced by Linus Pauling in the 1940s as part of his pioneering work on quantum chemistry. Since then, it has become a fundamental tool for chemists to describe and predict the properties of molecules.
Bond Order of CO32-
Calculating the bond order of the carbonate ion (CO32-) is essential for understanding its structure, bonding characteristics, and reactivity. Key aspects to consider include:
- Molecular Orbital Theory
- Resonance
- Hybridization
- Electronegativity
- Bond Length
- Bond Energy
- Quantum Chemistry
- Spectroscopy
- Density Functional Theory
- Computational Chemistry
These aspects are interconnected and provide a comprehensive understanding of the bond order in CO32-. For example, molecular orbital theory explains the formation of molecular orbitals and their interactions, while resonance describes the delocalization of electrons within the carbonate ion. Hybridization and electronegativity influence the bond lengths and bond energies, which are key factors in determining bond order. Spectroscopic techniques and computational chemistry provide experimental and theoretical insights into these aspects.
Molecular Orbital Theory
Molecular Orbital Theory provides a framework for understanding the electronic structure of molecules, including the carbonate ion (CO32-), and is essential for calculating its bond order. It describes the behavior of electrons in terms of molecular orbitals, which are mathematical functions that describe the wave-like behavior of electrons within a molecule.
- Atomic Orbitals: Electrons occupy atomic orbitals around individual atoms, but in molecules, these orbitals combine to form molecular orbitals.
- Linear Combination of Atomic Orbitals (LCAO): Molecular orbitals are constructed by combining atomic orbitals using mathematical equations. The number of molecular orbitals formed is equal to the number of atomic orbitals combined.
- Energy Levels: Molecular orbitals have specific energy levels, and electrons fill these orbitals in order of increasing energy, following the Aufbau principle.
- Bonding and Antibonding Orbitals: Molecular orbitals can be either bonding or antibonding. Bonding orbitals have lower energy and promote bonding between atoms, while antibonding orbitals have higher energy and weaken bonds.
By analyzing the molecular orbitals of CO32-, we can determine the number of bonding and antibonding electrons, which is crucial for calculating its bond order. Molecular Orbital Theory provides a powerful tool for understanding the electronic structure and bonding characteristics of molecules, including the carbonate ion.
Resonance
Resonance is a crucial concept in understanding the electronic structure and bonding of molecules, including the carbonate ion (CO32-). It describes the phenomenon where multiple Lewis structures can be drawn for a molecule, each representing a different arrangement of electrons. These resonance structures contribute to the overall electronic structure of the molecule, affecting its properties and reactivity.
In the case of CO32-, resonance plays a significant role in determining its bond order. The carbonate ion has three equivalent C-O bonds, but these bonds are not identical due to resonance. Resonance structures show that the negative charge on the carbonate ion is delocalized over all three oxygen atoms, resulting in an equal distribution of electron density among the C-O bonds. This delocalization lowers the energy of the molecule and increases its stability.
The concept of resonance is essential for accurately calculating the bond order of CO32-. By considering the resonance structures and the delocalization of electrons, we can determine the average bond order, which provides a more accurate representation of the bonding in the molecule. This understanding is crucial for predicting the reactivity and properties of CO32- in various chemical and biological systems.
Hybridization
Hybridization is a fundamental concept in chemistry that describes the mixing of atomic orbitals to form new hybrid orbitals with different shapes and energies. This process is crucial for understanding the bonding and molecular geometry of molecules, including the carbonate ion (CO32-). In the context of bond order calculations, hybridization plays a significant role in determining the number and type of bonds formed between atoms.
In the case of CO32-, the carbon atom undergoes sp2 hybridization, which involves the mixing of one 2s orbital and two 2p orbitals. This hybridization results in the formation of three equivalent sp2 hybrid orbitals that are arranged in a trigonal planar geometry. These hybrid orbitals overlap with the p orbitals of the three oxygen atoms, forming three sigma () bonds. The remaining p orbital on each oxygen atom overlaps with an sp2 hybrid orbital on the carbon atom, forming three pi () bonds. The combination of sigma and pi bonds contributes to the overall bond order of CO32-.
Understanding hybridization is essential for accurately calculating the bond order of CO32-. By considering the hybridization of the carbon atom and the overlap of atomic orbitals, we can determine the number of bonds formed and their relative strengths. This information is crucial for predicting the molecular geometry, stability, and reactivity of CO32- in various chemical and biological systems.
Electronegativity
Electronegativity is a fundamental property of atoms that describes their ability to attract electrons towards themselves within a chemical bond. In the context of calculating the bond order of the carbonate ion (CO32-), electronegativity plays a crucial role in determining the distribution of electrons and the strength of the bonds formed.
The electronegativity of an atom is influenced by its atomic number and the number of valence electrons. Generally, atoms with higher electronegativity values have a stronger attraction for electrons. In CO32-, the carbon atom has a higher electronegativity than the oxygen atoms. This difference in electronegativity leads to a polarization of the C-O bonds, with the electron density being drawn towards the oxygen atoms.
The electronegativity difference between the carbon and oxygen atoms affects the bond order of CO32-. A greater electronegativity difference results in a higher bond order, indicating a stronger bond. In the case of CO32-, the electronegativity difference between carbon and oxygen contributes to the strong C-O bonds and the overall stability of the carbonate ion.
Understanding the relationship between electronegativity and bond order is essential for predicting the properties and reactivity of molecules. By considering the electronegativity of the constituent atoms, chemists can gain insights into the distribution of electrons, bond strengths, and the overall molecular structure. This understanding is applied in various fields, including inorganic chemistry, materials science, and biochemistry, to design and optimize materials with desired properties.
Bond Length
Bond length, a crucial aspect of “how to calculate bond order of CO32-“, provides insights into the nature and strength of chemical bonds. It refers to the equilibrium distance between the nuclei of bonded atoms and is a fundamental property that influences various molecular properties and reactivity.
- Interatomic Distance: Bond length is directly related to the interatomic distance between the bonded atoms. Shorter bond lengths indicate stronger bonds, while longer bond lengths correspond to weaker bonds.
- Bond Order: Bond length is inversely proportional to bond order. Higher bond orders result in shorter bond lengths due to increased electron density and stronger attractive forces between the nuclei.
- Electronegativity: The electronegativity of atoms influences bond length. Bonds between atoms with large electronegativity differences tend to be shorter due to the stronger attraction of electrons towards the more electronegative atom.
- Hybridization: The hybridization of atomic orbitals affects bond length. Bonds formed by orbitals with more s-character (e.g., sp, sp2) are shorter than those formed by orbitals with more p-character (e.g., p, p2).
Understanding bond length is crucial for accurately calculating the bond order of CO32-. By considering the interatomic distances, electronegativity differences, and hybridization of the constituent atoms, chemists can gain valuable insights into the electronic structure and bonding characteristics of the carbonate ion. This knowledge is essential for predicting its stability, reactivity, and behavior in various chemical and biological systems.
Bond Energy
Bond energy, a fundamental aspect of “how to calculate bond order of CO32-“, represents the energy required to break a chemical bond between two atoms. It is a critical component in determining the stability and reactivity of molecules, providing valuable insights into the nature of chemical interactions.
Bond energy and bond order are closely related. Higher bond orders typically correspond to stronger bonds, which in turn have higher bond energies. This relationship arises from the increased electron density and stronger attractive forces between the nuclei in bonds with higher orders. By understanding the bond energy of CO32-, chemists can gain insights into the strength and stability of the carbonate ion, which is crucial for predicting its behavior in various chemical and biological systems.
For example, the strong C-O bonds in CO32-, characterized by high bond energies, contribute to the stability of the carbonate ion in aqueous solutions. This stability is essential for its role in regulating pH levels and participating in important biological processes, such as photosynthesis and respiration. Understanding the bond energy of CO32- enables researchers to design and optimize materials with desired properties, such as improved stability and reactivity, for applications in fields ranging from energy storage to biomedical engineering.
Quantum Chemistry
Quantum Chemistry provides a theoretical framework for studying the electronic structure of atoms and molecules, offering essential tools for calculating bond order and understanding chemical bonding in species like the carbonate ion (CO32-). It encompasses various concepts and techniques that enable researchers to analyze and predict molecular properties and reactivity.
- Wavefunction: The wavefunction, a mathematical function, describes the state of electrons in a molecule. By solving the Schrdinger equation, chemists can obtain the wavefunction and determine the electron distribution and energy levels.
- Molecular Orbitals: Quantum Chemistry introduces the concept of molecular orbitals, which are regions around the atomic nuclei where electrons are most likely to be found. These orbitals result from the combination of atomic orbitals and play a crucial role in determining chemical bonding.
- Electron Correlation: Electron correlation refers to the interactions between electrons in a molecule. Quantum Chemistry methods account for electron correlation, providing more accurate descriptions of molecular properties, including bond order.
- Density Functional Theory (DFT): DFT is a widely used technique in Quantum Chemistry that calculates the electron density in a molecule. From the electron density, various properties, including bond order, can be derived.
In the context of CO32-, Quantum Chemistry provides a powerful approach to calculate its bond order accurately. By considering the molecular orbitals, electron correlation, and electron density, chemists can gain insights into the nature of the C-O bonds and the overall electronic structure of the carbonate ion. This understanding is essential for predicting its stability, reactivity, and behavior in chemical and biological systems.
Spectroscopy
Spectroscopy plays a pivotal role in determining the bond order of the carbonate ion (CO32-). It involves the study of the interaction between electromagnetic radiation and matter, providing valuable insights into the electronic structure and bonding characteristics of molecules. By analyzing the absorption or emission of electromagnetic radiation, spectroscopists can obtain information about the energy levels, vibrational modes, and molecular composition of CO32-.
One of the most widely used spectroscopic techniques for calculating bond order is vibrational spectroscopy. By irradiating CO32- with infrared or Raman radiation, researchers can excite the vibrational modes of the molecule. The frequency and intensity of these vibrational bands provide information about the strength and type of bonds present. Stronger bonds typically exhibit higher vibrational frequencies, while weaker bonds have lower frequencies. By analyzing the vibrational spectra of CO32-, chemists can determine the bond order and gain insights into the molecular structure and dynamics.
Spectroscopy also finds applications in studying the electronic structure of CO32-. Techniques such as ultraviolet-visible (UV-Vis) and X-ray photoelectron spectroscopy (XPS) can provide information about the electronic transitions and oxidation states of the carbonate ion. These techniques help determine the energy levels of molecular orbitals and the distribution of electrons within the molecule, which are crucial for understanding the bond order and chemical reactivity of CO32-.
The practical applications of understanding the bond order of CO32- are diverse. In inorganic chemistry, it is essential for comprehending the stability and reactivity of carbonate-containing compounds, which are widely used in various industrial processes and environmental applications. In materials science, bond order calculations help design and optimize materials with specific properties, such as thermal stability and electrical conductivity. Furthermore, understanding the bond order of CO32- is crucial in biochemistry, where it plays a vital role in enzyme catalysis, ion transport, and biomineralization processes.
Density Functional Theory
Density Functional Theory (DFT) is a powerful computational quantum mechanical method widely used in chemistry and materials science to calculate the electronic structure of atoms, molecules, and solids. It plays a significant role in determining the bond order of molecules, including the carbonate ion (CO32-).
DFT is based on the Hohenberg-Kohn theorem, which states that the ground state energy of a system is uniquely determined by its electron density. This theorem provides the foundation for calculating the electronic structure and properties of molecules, including bond order, by approximating the electron density using various functionals.
In the context of CO32-, DFT calculations involve representing the electron density of the molecule using a specific functional. The choice of functional affects the accuracy of the calculated bond order. By optimizing the functional parameters, researchers can obtain more accurate electron densities and, consequently, more accurate bond orders.
Understanding the bond order of CO32- through DFT has practical applications in various fields. In inorganic chemistry, it helps predict the stability and reactivity of carbonate-containing compounds, which are used in industrial processes and environmental applications. In materials science, DFT calculations provide insights into the electronic structure and bonding of carbonate-based materials, enabling the design of materials with tailored properties. Additionally, in biochemistry, DFT is used to study the role of CO32- in enzyme catalysis and biomineralization processes.
Computational Chemistry
Computational Chemistry, a powerful tool in modern chemistry, plays a crucial role in determining the bond order of molecules, including the carbonate ion (CO32-). It involves using computational methods to solve quantum mechanical equations that describe the electronic structure of molecules.
As a critical component of “how to calculate bond order of CO32-“, Computational Chemistry provides accurate estimates of electron density distribution and energy levels within the molecule. By leveraging sophisticated algorithms and high-performance computing resources, researchers can model and analyze the behavior of electrons in CO32-, enabling them to determine the bond order and gain insights into the molecule’s chemical bonding characteristics.
Real-life examples of Computational Chemistry’s application in calculating the bond order of CO32- include using density functional theory (DFT) and Hartree-Fock (HF) methods. DFT is a widely used approach that approximates the electron density using a functional, while HF is a self-consistent field method that solves the Schrdinger equation iteratively. These methods provide quantitative results that help chemists understand the electronic structure and bonding in CO32-.
The practical applications of understanding the bond order of CO32- are diverse. In inorganic chemistry, it aids in predicting the stability and reactivity of carbonate-containing compounds, which are prevalent in various industrial processes and environmental applications. In materials science, it contributes to the design of novel materials with tailored properties, such as enhanced thermal stability and electrical conductivity. Furthermore, in biochemistry, it provides insights into enzyme catalysis and biomineralization processes involving CO32-.
Frequently Asked Questions
This section addresses common queries and clarifies concepts related to calculating the bond order of CO32-.
Question 1: What is bond order, and why is it important?
Answer: Bond order quantifies the strength and stability of chemical bonds. It provides insights into a molecule’s electronic structure, reactivity, and physical properties.
Question 2: How can I calculate the bond order of CO32-?
Answer: The bond order of CO32- can be determined using various methods, including molecular orbital theory, resonance theory, and computational chemistry.
Question 3: What factors influence the bond order of CO32-?
Answer: Factors such as hybridization, electronegativity, and the number of valence electrons affect the bond order of CO32-.
Question 4: How does bond order relate to bond length and bond energy?
Answer: Bond order is inversely proportional to bond length and directly proportional to bond energy. Stronger bonds, characterized by higher bond orders, have shorter bond lengths and higher bond energies.
Question 5: What practical applications does understanding bond order have?
Answer: Understanding bond order is crucial in various fields, including inorganic chemistry, materials science, and biochemistry. It helps predict compound stability, design new materials, and elucidate biological processes.
Question 6: How can I learn more about calculating bond order?
Answer: Refer to textbooks, scientific literature, or online resources for a deeper understanding of bond order calculations and their applications.
These FAQs provide a concise overview of key concepts related to calculating the bond order of CO32-. For further exploration, the following section delves into advanced topics and considerations in bond order calculations.
Tips for Calculating Bond Order of CO32-
This section provides practical tips and considerations for accurately calculating the bond order of the carbonate ion (CO32-).
Tip 1: Understand Molecular Orbital Theory: Gain a thorough understanding of molecular orbital theory, which provides the foundation for describing electron distribution and bonding in molecules.
Tip 2: Consider Resonance Structures: Take into account resonance structures to determine the delocalization of electrons and its impact on bond order.
Tip 3: Determine Hybridization: Identify the hybridization of the central carbon atom to understand the geometry and bonding characteristics of CO32-.
Tip 4: Account for Electronegativity: Consider the electronegativity differences between carbon and oxygen atoms to assess the polarization of bonds and its effect on bond order.
Tip 5: Utilize Spectroscopic Techniques: Employ spectroscopic methods, such as infrared or Raman spectroscopy, to obtain experimental data on bond vibrations and infer bond order.
Tip 6: Leverage Computational Chemistry: Use computational chemistry software to perform quantum mechanical calculations and accurately determine electron density distribution, which is crucial for bond order calculations.
Tip 7: Validate Results: Compare your calculated bond order with experimental data or values reported in scientific literature to ensure accuracy and reliability.
By following these tips, you can enhance your understanding of bond order calculations and gain valuable insights into the electronic structure and bonding characteristics of CO32-.
These tips pave the way for the concluding section, which will highlight the significance and applications of bond order calculations in various scientific disciplines.
Conclusion
Throughout this article, we have delved into the intricacies of calculating the bond order of the carbonate ion (CO32-), a versatile and ubiquitous species in chemistry and biology. By exploring concepts such as molecular orbital theory, resonance, hybridization, and electronegativity, we have gained a comprehensive understanding of the factors that govern bond order and its implications for the properties and reactivity of CO32-.
Key takeaways from our exploration include:
- Bond order provides valuable insights into the strength and stability of chemical bonds, enabling us to predict molecular properties and reactivity.
- Understanding bond order is crucial for fields such as inorganic chemistry, materials science, and biochemistry, where the behavior of carbonate-containing compounds plays a significant role.
- Accurate calculations of bond order require consideration of various factors, including molecular orbital interactions, resonance structures, and the interplay of electronegativity and hybridization.
As we continue to unravel the complexities of chemical bonding, the concept of bond order remains a fundamental tool for chemists and material scientists alike. By harnessing the power of computational chemistry and spectroscopic techniques, we can push the boundaries of our understanding and design novel materials with tailored properties for future technological advancements.
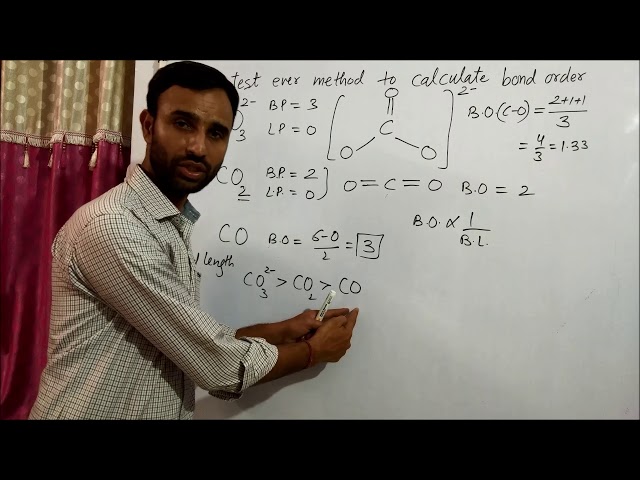