Bond order is a measure of the strength of a chemical bond between two atoms. It is calculated using the following formula:
Bond order = (Number of bonding electrons – Number of antibonding electrons) / 2
Hydrogen peroxide (H2O2) is a molecule that contains two hydrogen atoms and two oxygen atoms. The bond order of the O-O bond in H2O2 is 1. Bond order is an important concept in chemistry because it can be used to predict the properties of a molecule.
In this article, we will discuss how to calculate the bond order of H2O2. We will also explore the importance of bond order and its applications in chemistry.
How to Calculate Bond Order of H2O2
Bond order is a crucial concept in chemistry, providing insights into the strength and nature of chemical bonds. Understanding how to calculate bond order is essential for comprehending molecular structures and properties.
- Molecular Orbital Theory
- Bonding and Antibonding Electrons
- Resonance Structures
- Hybridization
- Electronegativity
- Bond Length
- Bond Energy
- Magnetic Properties
- Spectroscopic Data
- Quantum Mechanics
These aspects collectively contribute to the calculation and interpretation of bond order, offering valuable insights into the electronic structure and chemical behavior of molecules. By considering these factors, chemists can gain a deeper understanding of molecular bonding and its implications in various chemical systems.
Molecular Orbital Theory
Molecular Orbital Theory (MOT) is a fundamental theory in quantum chemistry that describes the electronic structure of molecules. It provides a mathematical framework for understanding how electrons are distributed within a molecule and how this distribution affects the molecule’s properties.
MOT is critical for understanding how to calculate bond order, as it provides the theoretical basis for determining the number of bonding and antibonding electrons in a molecule. The bond order, in turn, is a measure of the strength and stability of the bond between two atoms.
In the case of H2O2, MOT can be used to calculate the bond order of the O-O bond. The O-O bond in H2O2 is a single bond, meaning that there is one bonding electron pair between the two oxygen atoms. This can be determined using MOT by examining the molecular orbitals of H2O2 and identifying the number of electrons that occupy the bonding and antibonding orbitals.
The practical applications of understanding the connection between MOT and bond order are numerous. For example, MOT can be used to predict the stability of molecules, design new materials with specific properties, and understand the mechanisms of chemical reactions.
Bonding and Antibonding Electrons
Bonding and antibonding electrons play a fundamental role in determining the bond order of H2O2. Bonding electrons are those that occupy molecular orbitals that are symmetric with respect to the internuclear axis, leading to constructive interference and increased electron density between the nuclei. Antibonding electrons, on the other hand, occupy molecular orbitals that are antisymmetric with respect to the internuclear axis, resulting in destructive interference and decreased electron density between the nuclei.
- Molecular Orbital Symmetry
The symmetry of molecular orbitals determines whether electrons are bonding or antibonding. Bonding orbitals have symmetric wavefunctions, while antibonding orbitals have antisymmetric wavefunctions.
- Electron Density
Bonding electrons increase the electron density between the nuclei, strengthening the bond. Antibonding electrons decrease the electron density between the nuclei, weakening the bond.
- Bond Order
The bond order is directly related to the number of bonding and antibonding electrons. A higher bond order indicates a stronger bond.
- H2O2 Example
In H2O2, there are two bonding electrons and two antibonding electrons in the O-O bond. This results in a bond order of 1, indicating a single bond.
Understanding bonding and antibonding electrons is crucial for calculating bond order and predicting the properties of molecules. By considering the symmetry of molecular orbitals and the resulting electron density, chemists can gain valuable insights into the nature of chemical bonds.
Resonance Structures
Resonance structures are a powerful tool for representing the electronic structure of molecules. They are particularly useful for molecules that have multiple bonds or lone pairs of electrons, such as H2O2. Resonance structures can provide valuable insights into the bond order and other properties of molecules.
- Equivalent Lewis Structures
Resonance structures are different Lewis structures that represent the same molecule. They have the same number of atoms and electrons, but the atoms are arranged differently.
- Delocalized Electrons
The electrons in a resonance structure are not localized to a single atom or bond. Instead, they are delocalized over the entire molecule.
- Resonance Hybrid
The actual structure of a molecule that has resonance structures is a resonance hybrid. The resonance hybrid is a weighted average of the resonance structures.
- Bond Order
The bond order in a resonance hybrid is an average of the bond orders in the resonance structures. For example, the O-O bond order in H2O2 is 1.5, which is an average of the 1 and 2 bond orders in the two resonance structures.
Resonance structures are an important tool for understanding the electronic structure and properties of molecules. They can be used to calculate bond orders, predict molecular shapes, and explain chemical reactivity.
Hybridization
Hybridization is a fundamental concept in chemistry that describes the mixing of atomic orbitals to form new hybrid orbitals with different shapes and energies. It plays a crucial role in determining the molecular geometry and properties of compounds, including the bond order of H2O2.
In the case of H2O2, the oxygen atoms undergo sp3 hybridization. This means that one 2s orbital and three 2p orbitals from each oxygen atom combine to form four equivalent sp3 hybrid orbitals. These hybrid orbitals then overlap to form the two O-H bonds and the O-O bond.
The hybridization of the oxygen atoms affects the bond order of the O-O bond. The sp3 hybrid orbitals have a more significant overlap than pure p orbitals, resulting in a stronger O-O bond. This is reflected in the bond order of 1.5, which indicates a bond that is stronger than a single bond but weaker than a double bond.
Understanding the connection between hybridization and bond order is essential for accurately predicting the properties of molecules. Hybridization can also be used to explain a wide range of chemical phenomena, such as the shapes of molecules, the strengths of bonds, and the reactivity of molecules.
Electronegativity
Electronegativity is a crucial concept in chemistry that measures the ability of an atom to attract electrons towards itself. It plays a significant role in determining the bond order of H2O2 and understanding its molecular properties.
- Pauling Scale
The Pauling scale is a widely used method for quantifying electronegativity. It assigns numerical values to elements based on their ability to attract electrons.
- Electronegativity and Bond Order
The difference in electronegativity between two atoms influences the bond order. A greater difference in electronegativity leads to a more polar bond and a higher bond order.
- H2O2 Example
In H2O2, the electronegativity of oxygen (3.44) is higher than that of hydrogen (2.20). This difference in electronegativity results in a polar O-H bond and a higher bond order of 1.5.
- Applications
Electronegativity is used to predict bond types, molecular geometry, and chemical reactivity. It is a fundamental property that helps us understand and manipulate chemical systems.
In summary, electronegativity is a key factor in determining the bond order of H2O2. The Pauling scale provides a quantitative measure of electronegativity, which can be used to predict the polarity and strength of chemical bonds. Understanding electronegativity is essential for comprehending the structure and properties of molecules.
Bond Length
Bond length is a critical component of how to calculate bond order of h2o2. It is defined as the distance between the nuclei of two bonded atoms. Bond length is inversely related to bond order; as bond order increases, bond length decreases. This relationship is due to the fact that stronger bonds (higher bond order) result in a greater overlap of electron orbitals, which leads to a shorter bond length.
In the case of h2o2, the bond order of the O-O bond is 1.5. This indicates that the O-O bond is stronger than a single bond but weaker than a double bond. The bond length of the O-O bond is 1.48 , which is shorter than the bond length of a typical single bond (1.54 ) but longer than the bond length of a typical double bond (1.21 ). This is consistent with the bond order of 1.5.
The relationship between bond length and bond order has important implications for understanding the structure and properties of molecules. For example, bond length can be used to predict the strength of a chemical bond, the molecular geometry, and the reactivity of a molecule. Bond length is also a key parameter in computational chemistry, where it is used to develop models of molecular structures and to predict the properties of new materials.
Bond Energy
Bond energy is a fundamental concept in chemistry that measures the strength of a chemical bond. It plays a crucial role in determining the bond order of H2O2 and understanding its molecular properties.
- Bond Dissociation Energy
Bond dissociation energy is the energy required to break a bond between two atoms. It is a direct measure of bond strength.
- Bond Length and Bond Energy
Bond length is inversely related to bond energy. Stronger bonds have shorter bond lengths, and vice versa.
- Resonance and Bond Energy
Resonance structures can affect bond energy. Resonance structures with more delocalized electrons generally have stronger bonds.
- Bond Order and Bond Energy
Bond order is directly related to bond energy. Higher bond orders indicate stronger bonds.
Understanding bond energy is essential for comprehending the structure and properties of molecules. Bond energy can be used to predict the stability of molecules, design new materials with specific properties, and understand the mechanisms of chemical reactions.
Magnetic Properties
Magnetic properties are closely related to the bond order of a molecule. Bond order is a measure of the strength of a chemical bond, and it can be used to predict the magnetic properties of a molecule. For example, molecules with higher bond orders are typically more diamagnetic, while molecules with lower bond orders are typically more paramagnetic.
In the case of H2O2, the bond order of the O-O bond is 1.5. This indicates that the O-O bond is stronger than a single bond, but weaker than a double bond. As a result, H2O2 is diamagnetic.
The magnetic properties of H2O2 can be used to understand its chemical reactivity. For example, H2O2 is a strong oxidizing agent, and its diamagnetic nature indicates that it is not easily reduced. This makes H2O2 a useful reagent for a variety of chemical reactions.
In summary, the magnetic properties of H2O2 are closely related to its bond order. This relationship can be used to understand the chemical reactivity of H2O2 and to design new materials with specific magnetic properties.
Spectroscopic Data
Spectroscopic data plays a crucial role in determining the bond order of H2O2. Spectroscopy is the study of the absorption and emission of electromagnetic radiation by matter, and it provides valuable insights into the molecular structure and bonding of compounds. In the case of H2O2, spectroscopic data can be used to determine the vibrational frequencies of the O-O bond, which are directly related to the bond order.
One of the most important spectroscopic techniques used to study H2O2 is infrared spectroscopy. Infrared radiation can be absorbed by molecules when it matches the vibrational frequency of a particular bond. The frequency of the absorbed radiation corresponds to the energy difference between the ground and excited vibrational states of the bond. By measuring the infrared spectrum of H2O2, it is possible to determine the vibrational frequency of the O-O bond and hence the bond order.
Spectroscopic data can also be used to study other aspects of the bonding in H2O2, such as the hybridization of the oxygen atoms and the presence of resonance structures. This information can provide a more complete understanding of the electronic structure of H2O2 and its chemical properties.
In summary, spectroscopic data is a critical component of how to calculate bond order of h2o2. It provides valuable insights into the molecular structure and bonding of compounds, and it can be used to determine the vibrational frequencies, hybridization, and resonance structures of H2O2. This information is essential for understanding the chemical properties of H2O2 and its applications in various fields.
Quantum Mechanics
Quantum mechanics provides the theoretical framework for understanding the electronic structure of atoms and molecules, which is essential for calculating bond order. It offers a set of mathematical tools and concepts that describe the behavior of electrons and their interactions with atomic nuclei.
- Wave-Particle Duality
Quantum mechanics recognizes the dual nature of electrons as both particles and waves. This concept is crucial for understanding the formation of molecular orbitals and the distribution of electrons within them.
- Schrdinger Equation
The Schrdinger equation is a mathematical equation that describes the wavefunction of an electron. The wavefunction contains information about the electron’s energy, momentum, and spatial distribution, allowing for the calculation of electron density and bond order.
- Molecular Orbital Theory
Molecular orbital theory uses quantum mechanics to describe the electronic structure of molecules. It provides a framework for understanding how atomic orbitals combine to form molecular orbitals, which in turn determine the bond order and other properties of the molecule.
- Computational Chemistry
Computational chemistry methods, such as density functional theory (DFT), employ quantum mechanics to calculate the electronic structure and properties of molecules. These methods can be used to determine bond orders, predict molecular geometries, and simulate chemical reactions.
Quantum mechanics is a powerful tool for understanding and calculating bond order in H2O2 and other molecules. It provides a theoretical basis for interpreting experimental data and predicting the properties of molecules, enabling researchers to gain insights into chemical bonding and molecular behavior.
Frequently Asked Questions
This section addresses common questions and provides additional clarifications on various aspects of calculating bond order in H2O2.
Question 1: What is the significance of bond order in H2O2?
Answer: Bond order determines the strength and stability of the O-O bond, influencing the molecule’s physical and chemical properties, such as reactivity and spectroscopic behavior.
Question 2: How does resonance affect the bond order of H2O2?
Answer: Resonance structures contribute to the delocalization of electrons, leading to an average bond order that reflects the combined contributions of all resonance forms.
Question 3: Can electronegativity influence the bond order in H2O2?
Answer: Electronegativity differences between oxygen and hydrogen atoms contribute to the polarity of the O-H bonds and indirectly affect the overall bond order of H2O2.
Question 4: How is bond order related to bond length and bond energy?
Answer: Bond order is inversely proportional to bond length; stronger bonds (higher bond order) correspond to shorter bond lengths and higher bond energies.
Question 5: What experimental techniques can be used to determine the bond order of H2O2?
Answer: Infrared spectroscopy, Raman spectroscopy, and X-ray crystallography are commonly employed experimental methods for determining bond order based on vibrational frequencies, bond lengths, and molecular structure.
Question 6: How does quantum mechanics contribute to our understanding of bond order in H2O2?
Answer: Quantum mechanics provides the theoretical framework for describing electron behavior and molecular orbital formation, enabling the calculation of bond order through methods like molecular orbital theory and density functional theory (DFT).
These FAQs provide a comprehensive overview of essential concepts related to bond order calculation in H2O2. Understanding these concepts is crucial for
In the next section, we will explore advanced applications of bond order calculations in H2O2 and other molecules, including its implications for chemical synthesis, materials design, and biological systems.
Tips for Calculating Bond Order of H2O2
To enhance your understanding and accuracy when calculating the bond order of H2O2, consider the following practical tips:
Tip 1: Utilize molecular orbital theory to determine the number of bonding and antibonding electrons, which directly influence bond order.
Tip 2: Examine resonance structures to account for delocalization of electrons, which can affect the overall bond order.
Tip 3: Consider electronegativity differences between atoms to assess the polarity of bonds and its impact on bond order.
Tip 4: Measure bond lengths experimentally to infer bond order, as stronger bonds typically exhibit shorter bond lengths.
Tip 5: Analyze bond energies to evaluate bond strength, with higher bond energies corresponding to higher bond orders.
Tip 6: Employ spectroscopic techniques, such as infrared spectroscopy, to determine vibrational frequencies, which can provide insights into bond order.
Tip 7: Utilize computational chemistry methods, like density functional theory, to calculate bond order through quantum mechanical simulations.
Tip 8: Validate your bond order calculations by comparing them with experimental data or literature values to ensure accuracy.
By implementing these tips, you can improve the precision and depth of your bond order calculations for H2O2, leading to a more comprehensive understanding of its molecular structure and properties.
In the final section of this article, we will explore advanced applications of bond order calculations in H2O2 and other molecules, demonstrating their significance in various scientific fields.
Conclusion
In this article, we have explored the various methods for calculating the bond order of H2O2, providing a comprehensive overview of the concept and its significance in understanding the molecular structure and properties of H2O2. Key points discussed include the use of molecular orbital theory, resonance structures, electronegativity, and spectroscopic techniques, emphasizing the interconnectedness of these approaches in providing a complete picture of bond order.
Understanding bond order is not only crucial for H2O2 but also extends to other molecules, offering insights into their stability, reactivity, and various applications. This knowledge has far-reaching implications in fields such as chemistry, materials science, and biochemistry, driving the development of new materials, pharmaceuticals, and energy sources. As we continue to delve deeper into the realm of molecular bonding, the calculation of bond order will remain a fundamental tool, empowering researchers to unravel the mysteries of molecular behavior and design novel compounds with tailored properties.
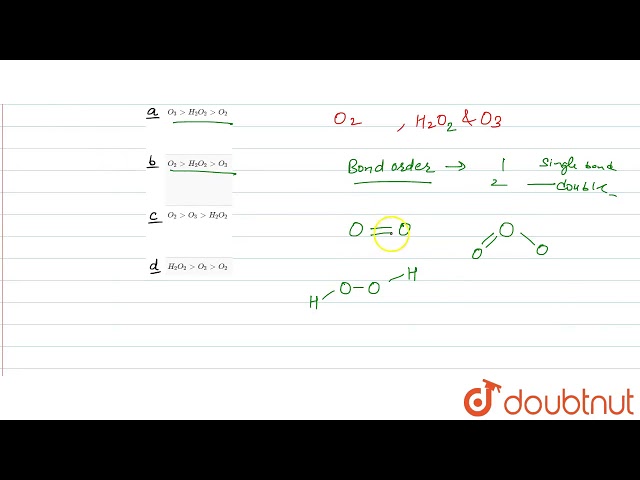