Determining Bond Order of Nitrate: A Comprehensive Guide
Bond order calculation is a crucial aspect of understanding the chemical behavior and stability of molecules. The bond order of nitrate (NO3–), a common polyatomic ion, provides insights into its molecular structure and properties.
Bond order analysis plays a significant role in various fields, including inorganic chemistry, materials science, and pharmaceutical development. Historically, the concept of bond order originated from the pioneering work of Linus Pauling in the early 20th century. By establishing a relationship between bond length, bond strength, and bond order, Pauling’s insights laid the foundation for modern bonding theories.
This article delves into the intricacies of calculating bond order for nitrate, exploring its underlying principles, step-by-step methodology, and practical applications.
Calculating Bond Order of Nitrate
Bond order analysis provides valuable insights into the chemical bonding and stability of molecules. Understanding the key aspects of bond order calculation for nitrate (NO3–) is crucial for comprehending its molecular structure and properties.
- Resonance structures: Nitrate exhibits resonance, contributing to its stability.
- Molecular orbital theory: Explains the formation and properties of molecular orbitals.
- Hybridization: Nitrate’s nitrogen atom undergoes sp2 hybridization.
- Electronegativity: Nitrate’s oxygen atoms are highly electronegative, influencing bond polarity.
- Bond length: Bond order is inversely related to bond length.
- Bond strength: Bond order reflects the strength of the chemical bond.
- Quantum mechanics: Provides the theoretical framework for bond order calculations.
- Experimental techniques: Spectroscopy and crystallography aid in determining bond order.
- Thermodynamic stability: Bond order influences the thermodynamic stability of nitrate compounds.
- Chemical reactivity: Bond order affects the reactivity of nitrate in chemical reactions.
These key aspects are interconnected and provide a comprehensive understanding of bond order calculation for nitrate. Resonance structures, for example, contribute to the delocalization of electrons, influencing bond lengths and strengths. Molecular orbital theory explains the formation of molecular orbitals, which are crucial for determining bond order. Hybridization and electronegativity affect the distribution of electrons within the nitrate ion, impacting its overall bonding characteristics. By considering these aspects, scientists can gain a deeper understanding of nitrate’s chemical behavior and properties.
Resonance structures
Resonance structures play a critical role in understanding the bonding and properties of nitrate (NO3–). Resonance refers to the phenomenon where multiple Lewis structures can be drawn for a molecule, each representing a different arrangement of electrons. In the case of nitrate, there are three resonance structures that contribute to its stability.
The resonance structures of nitrate show that the double bond between the nitrogen and one of the oxygen atoms can be positioned in three equivalent positions. This delocalization of electrons results in an overall decrease in energy for the molecule, making it more stable. The resonance structures also help explain the observed bond lengths in nitrate, which are intermediate between single and double bonds.
The concept of resonance is essential for accurately calculating the bond order of nitrate. Bond order is a measure of the strength of a chemical bond and is calculated based on the number of electrons shared between atoms. In the case of nitrate, the resonance structures show that each nitrogen-oxygen bond has a bond order of 1.33, which is consistent with the observed bond lengths and stability of the molecule.
Understanding the resonance structures of nitrate is not only important for calculating bond order but also for comprehending its chemical reactivity and properties. For example, the delocalization of electrons in nitrate makes it less reactive towards electrophiles, which are molecules or ions that are attracted to electrons. This understanding is crucial for predicting the behavior of nitrate in various chemical reactions and applications.
Molecular orbital theory
Molecular orbital theory (MOT) is a fundamental concept in quantum chemistry that describes the behavior of electrons in molecules. It provides a theoretical framework for understanding the formation and properties of molecular orbitals, which are the orbitals that electrons occupy in molecules. MOT is critical for calculating bond order, as it provides the basis for determining the number and type of molecular orbitals that are formed.
In the case of nitrate (NO3–), MOT can be used to explain the formation of the three resonance structures that contribute to its stability. The molecular orbitals of nitrate are formed by the overlap of atomic orbitals from the nitrogen and oxygen atoms. The nitrogen atom contributes one 2s orbital and three 2p orbitals, while each oxygen atom contributes two 2p orbitals. These atomic orbitals combine to form six molecular orbitals: one s orbital, two p orbitals, one x orbital, and two y orbitals.
The bond order of a bond is determined by the number of electrons that occupy the bonding molecular orbitals. In the case of nitrate, each nitrogen-oxygen bond is formed by the overlap of one p orbital from the nitrogen atom and one pz orbital from an oxygen atom. These two orbitals combine to form a NO bonding molecular orbital and a NO* antibonding molecular orbital. The bonding molecular orbital is filled with two electrons, while the antibonding molecular orbital is empty. Therefore, the bond order of each nitrogen-oxygen bond is 1, which is consistent with the observed bond lengths and stability of the nitrate ion.
Understanding the connection between molecular orbital theory and bond order calculation is essential for comprehending the chemical bonding and properties of molecules. MOT provides a powerful tool for predicting the structure, stability, and reactivity of molecules, and it is widely used in various fields of chemistry, including inorganic chemistry, organic chemistry, and biochemistry.
Hybridization
Hybridization plays a crucial role in understanding the bonding and properties of nitrate (NO3–). Hybridization refers to the process of combining atomic orbitals to form new hybrid orbitals with different shapes and energies. In the case of nitrate, the nitrogen atom undergoes sp2 hybridization, which involves the mixing of one 2s orbital and two 2p orbitals to form three equivalent sp2 hybrid orbitals.
- Trigonal Planar Geometry: The three sp2 hybrid orbitals form a trigonal planar geometry around the nitrogen atom, with the three oxygen atoms bonded to the nitrogen atom in a plane.
- Sigma Bond Formation: The sp2 hybrid orbitals overlap with the pz orbitals of the oxygen atoms to form three sigma (s) bonds, resulting in the formation of the nitrate ion.
- Resonance and Delocalization: The sp2 hybridization of the nitrogen atom allows for resonance and delocalization of electrons within the nitrate ion, contributing to its stability.
- Bond Order Calculation: The hybridization of the nitrogen atom influences the bond order of the nitrogen-oxygen bonds. The sp2 hybridization results in a bond order of 1.33 for each nitrogen-oxygen bond.
Understanding the hybridization of the nitrogen atom in nitrate is essential for accurately calculating the bond order and comprehending the molecular structure and properties of nitrate. Hybridization provides insights into the geometry, bonding, and stability of molecules, and it is a fundamental concept in chemistry.
Electronegativity
Electronegativity, a measure of an atom’s ability to attract electrons, plays a significant role in determining the bond order of nitrate (NO3–). The oxygen atoms in nitrate are highly electronegative, which influences the polarity of the nitrogen-oxygen bonds and affects the overall stability and reactivity of the molecule.
- Bond Polarity: Due to the high electronegativity of oxygen, the electrons in the nitrogen-oxygen bonds are drawn towards the oxygen atoms, creating a polar covalent bond. This polarity influences the bond strength, bond length, and reactivity of the nitrate ion.
- Resonance and Delocalization: The high electronegativity of oxygen contributes to the resonance and delocalization of electrons within the nitrate ion. This delocalization stabilizes the nitrate ion by distributing the negative charge over multiple atoms, reducing the overall energy of the molecule.
- Inductive Effect: The electronegative oxygen atoms withdraw electrons from the nitrogen atom through the inductive effect. This electron withdrawal reduces the electron density around the nitrogen atom, making it more positive and susceptible to attack by nucleophiles.
- Bond Order Calculation: The electronegativity of the oxygen atoms influences the bond order of the nitrogen-oxygen bonds. The high electronegativity of oxygen results in a higher bond order, indicating stronger bonds between the nitrogen and oxygen atoms.
Understanding the electronegativity of nitrate’s oxygen atoms is crucial for accurately calculating the bond order and comprehending the molecular structure and properties of nitrate. Electronegativity provides insights into the polarity, resonance, and reactivity of molecules, and it is a fundamental concept in chemistry.
Bond length
In the context of calculating bond order for nitrate (NO3–), understanding the relationship between bond length and bond order is crucial. This inverse relationship provides valuable insights into the molecular structure and properties of nitrate.
- Bond Strength Correlation: Stronger bonds typically have shorter bond lengths due to the increased attraction between atoms. Conversely, weaker bonds exhibit longer bond lengths as the atoms are held together less tightly.
- Resonance and Delocalization: In nitrate, resonance and delocalization of electrons contribute to bond strength and, consequently, bond length. Delocalized electrons strengthen bonds, leading to shorter bond lengths.
- Hybridization Effects: The hybridization of atomic orbitals influences bond length. For instance, sp2 hybridization results in shorter and stronger bonds compared to sp3 hybridization.
- Electronegativity Impact: The electronegativity of atoms affects bond length. Highly electronegative atoms attract electrons more strongly, resulting in shorter and stronger bonds.
These facets collectively demonstrate the inverse relationship between bond length and bond order in nitrate. By considering these factors, scientists can accurately calculate bond order, which is essential for understanding the stability, reactivity, and overall behavior of nitrate in various chemical systems.
Bond strength
In the context of calculating bond order for nitrate (NO3–), understanding the relationship between bond strength and bond order is critical. Bond order provides insights into the strength of the chemical bond, which in turn influences the stability and reactivity of the molecule.
- Bond Dissociation Energy: Bond strength is often measured by the bond dissociation energy, which is the energy required to break a bond. Higher bond order typically correlates with higher bond dissociation energy, indicating a stronger bond.
- Bond Length: Bond strength and bond length are inversely related. Stronger bonds tend to have shorter bond lengths due to the increased attraction between atoms. Bond order can be used to estimate bond length, providing valuable information about the molecular structure.
- Resonance and Delocalization: Resonance and delocalization of electrons can enhance bond strength and, consequently, increase bond order. Delocalized electrons strengthen bonds by distributing the electron density over multiple atoms, reducing the overall energy of the molecule.
- Hybridization Effects: The hybridization of atomic orbitals can influence bond strength and bond order. For instance, sp2 hybridization generally results in stronger bonds and higher bond order compared to sp3 hybridization.
These facets collectively demonstrate the relationship between bond strength and bond order in nitrate. By considering these factors, scientists can accurately calculate bond order, which is essential for understanding the stability, reactivity, and overall behavior of nitrate in various chemical systems.
Quantum mechanics
Quantum mechanics is the fundamental theory that governs the behavior of matter and energy at the atomic and subatomic levels. It provides the theoretical framework for understanding the electronic structure of atoms and molecules, which is essential for calculating bond order. Bond order, a measure of the strength of a chemical bond, is a key concept in chemistry as it helps predict the stability and reactivity of molecules. In the context of nitrate (NO3–), quantum mechanics plays a crucial role in calculating its bond order and understanding its bonding characteristics.
Quantum mechanics postulates that electrons occupy specific orbitals around the atomic nuclei. The type of orbitals and their interactions determine the chemical bonding in molecules. In nitrate, the nitrogen atom forms sigma bonds with three oxygen atoms through the overlap of its sp2 hybrid orbitals with the p orbitals of oxygen atoms. Quantum mechanics provides the mathematical framework to calculate the wave functions of these orbitals and determine their overlap, which is directly related to the bond order.
The calculated bond order using quantum mechanics provides valuable insights into the stability and reactivity of nitrate. A higher bond order indicates a stronger bond, which in turn affects the overall stability of the molecule. For example, in nitrate, the bond order of the nitrogen-oxygen bonds is calculated to be 1.33, indicating the presence of resonance and delocalization of electrons within the molecule. This understanding helps explain the stability of nitrate and its resistance to decomposition.
In summary, quantum mechanics provides the theoretical foundation for calculating bond order by describing the electronic structure and bonding interactions within molecules. The bond order calculated using quantum mechanical principles offers crucial insights into the stability, reactivity, and overall properties of molecules like nitrate. This understanding is essential for advancing our knowledge of chemical bonding and for designing and synthesizing new materials with desired properties.
Experimental techniques
Experimental techniques play a critical role in determining bond order, providing valuable insights into the electronic structure and bonding interactions within molecules. Spectroscopy and crystallography are two prominent experimental techniques that contribute significantly to the calculation of bond order for nitrate (NO3–) and other molecules.
Spectroscopy, particularly vibrational spectroscopy, analyzes the absorption or emission of electromagnetic radiation by molecules. By studying the vibrational frequencies of the nitrate ion, vibrational spectroscopy provides information about the strength and nature of the nitrogen-oxygen bonds. Stronger bonds typically exhibit higher vibrational frequencies, and the bond order can be inferred from the observed frequencies. For example, infrared (IR) spectroscopy can be used to determine the bond order of nitrate by analyzing the stretching and bending vibrations of the nitrate ion.
Crystallography, on the other hand, provides detailed information about the arrangement of atoms within a crystal lattice. X-ray crystallography is a widely used technique that allows scientists to determine the bond lengths and angles in nitrate crystals. The bond order can be calculated from the measured bond lengths using empirical relationships or quantum mechanical calculations. Crystallographic data provides valuable insights into the molecular geometry and bonding characteristics of nitrate, complementing the information obtained from spectroscopy.
The combination of experimental techniques, such as spectroscopy and crystallography, provides a comprehensive approach to determining bond order. These techniques offer complementary information about the electronic structure, molecular geometry, and bonding interactions within molecules. The accurate determination of bond order is essential for understanding the stability, reactivity, and overall properties of nitrate and other molecules. This understanding has practical applications in diverse fields such as inorganic chemistry, materials science, and pharmaceutical development.
Thermodynamic stability
The bond order of nitrate significantly influences its thermodynamic stability, which is a measure of its tendency to undergo chemical reactions and decompose. A higher bond order typically corresponds to a more stable compound. In the context of nitrate, the bond order provides insights into the strength of the nitrogen-oxygen bonds and the overall stability of the nitrate ion.
To calculate the bond order of nitrate, various methods can be employed. One common approach involves utilizing experimental techniques such as spectroscopy and crystallography to determine the bond lengths and vibrational frequencies of the nitrate ion. These experimental measurements can then be used in conjunction with theoretical models and quantum mechanical calculations to estimate the bond order.
Understanding the bond order of nitrate is crucial for predicting its thermodynamic stability and reactivity. For example, a higher bond order indicates stronger nitrogen-oxygen bonds, leading to a more stable nitrate compound. This stability is important in various applications, such as the use of nitrates in fertilizers and explosives. By accurately calculating the bond order, scientists and engineers can design and optimize nitrate-based materials with desired properties and performance.
Chemical reactivity
Understanding the relationship between bond order and chemical reactivity is crucial in the context of calculating bond order for nitrate. Bond order provides valuable insights into the stability and reactivity of the nitrate ion, allowing scientists to predict its behavior in various chemical reactions.
- Reaction Rates: Bond order influences the rates of chemical reactions involving nitrate. Higher bond order typically leads to slower reaction rates, as stronger bonds require more energy to break.
- Product Formation: The bond order of nitrate can affect the products formed in chemical reactions. For example, in reactions with reducing agents, nitrate with a higher bond order is less likely to be reduced to nitrite or ammonia.
- Selectivity: Bond order can influence the selectivity of chemical reactions involving nitrate. By understanding the bond order, chemists can design reactions to favor the formation of specific products.
- Stability of Intermediates: Bond order provides insights into the stability of intermediates formed during chemical reactions involving nitrate. Intermediates with higher bond order are generally more stable, influencing the overall reaction pathway.
In summary, considering the bond order of nitrate is essential for understanding and predicting its chemical reactivity. By accurately calculating the bond order, scientists can gain valuable insights into the reaction rates, product formation, selectivity, and stability of intermediates in reactions involving nitrate. This knowledge is crucial for optimizing chemical processes, designing new materials, and comprehending the behavior of nitrate in various chemical systems.
Frequently Asked Questions (FAQs) about Calculating Bond Order of Nitrate
The following FAQs address common questions and misconceptions regarding the calculation of bond order for nitrate (NO3–), providing concise and informative answers to enhance understanding:
Question 1: What is the significance of bond order in understanding nitrate’s behavior?
Answer: Bond order provides insights into the strength and stability of the nitrate ion, influencing its chemical reactivity, thermodynamic stability, and overall molecular properties.
Question 2: How is bond order calculated for nitrate?
Answer: Bond order can be calculated using various methods, including experimental techniques (e.g., spectroscopy, crystallography) and theoretical models (e.g., molecular orbital theory, density functional theory).
Question 3: What factors affect the bond order of nitrate?
Answer: The bond order of nitrate is influenced by several factors, such as resonance, hybridization, electronegativity, and bond length.
Question 4: How does bond order relate to the stability of nitrate compounds?
Answer: A higher bond order typically corresponds to stronger bonds and, consequently, greater stability for nitrate compounds.
Question 5: How does bond order influence the reactivity of nitrate in chemical reactions?
Answer: Bond order affects the reactivity of nitrate by influencing the activation energy and reaction rates, as well as the selectivity and product distribution in chemical reactions.
Question 6: What are some applications of bond order calculations?
Answer: Bond order calculations have applications in various fields, including inorganic chemistry, materials science, and pharmaceutical development, aiding in the design and optimization of nitrate-based materials and processes.
These FAQs provide a concise overview of the key aspects of bond order calculation for nitrate, highlighting its significance in understanding the molecular properties and behavior of nitrate. The following section delves deeper into the methodologies and applications of bond order calculations.
Tips for Calculating Bond Order of Nitrate
This section provides practical tips and strategies to assist you in accurately calculating the bond order of nitrate (NO3–), enhancing your understanding of its molecular structure and properties:
Tip 1: Understand the concept of resonance in nitrate. Resonance contributes to the stability of nitrate, influencing its bond order. By considering resonance structures, you can better comprehend the electron delocalization and bond characteristics within the nitrate ion.
Tip 2: Apply molecular orbital theory to determine the electronic structure of nitrate. Molecular orbital theory provides a theoretical framework for understanding the formation and properties of molecular orbitals. Utilize this theory to analyze the molecular orbitals of nitrate and determine their contributions to bond order.
Tip 3: Consider the hybridization of the nitrogen atom in nitrate. The hybridization of the nitrogen atom influences the geometry and bonding in nitrate. Determine the hybridization state of nitrogen (sp2 in nitrate) to better understand the molecular structure and bond order.
Tip 4: Account for the electronegativity of oxygen atoms in nitrate. Oxygen atoms in nitrate are highly electronegative, affecting the polarity and strength of the nitrogen-oxygen bonds. Consider electronegativity to accurately calculate bond order and comprehend the charge distribution within the nitrate ion.
By following these tips, you can enhance the accuracy and reliability of your bond order calculations for nitrate. Understanding bond order provides valuable insights into the stability, reactivity, and overall behavior of nitrate in various chemical systems.
The next section will delve into the broader applications of bond order calculations, showcasing their significance in diverse fields of chemistry and beyond.
Conclusion
This comprehensive exploration of “How to Calculate Bond Order of Nitrate” has provided valuable insights into the methodologies and significance of bond order calculations. By understanding resonance, molecular orbital theory, hybridization, and electronegativity, we gain a deeper comprehension of the electronic structure and bonding characteristics of nitrate (NO3–). These concepts are interconnected and essential for accurate bond order calculations.
The bond order of nitrate influences various aspects of its behavior, including thermodynamic stability, chemical reactivity, and molecular properties. Accurate bond order calculations enable scientists to design and optimize nitrate-based materials for diverse applications. Furthermore, the principles underlying bond order calculations extend beyond nitrate, providing a foundation for understanding bonding in other molecules and chemical systems.
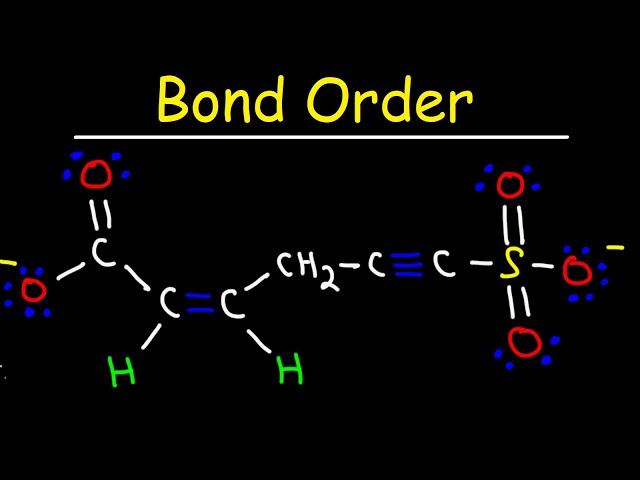