Bond order is a crucial concept in chemistry, representing the strength and nature of chemical bonds between atoms. Understanding how to calculate bond order of NO2 helps us determine the stability and reactivity of this important molecule. In chemistry, bond order provides insights into a molecule’s electronic structure and influences its physical and chemical properties.
Calculating bond order is essential for studying reaction mechanisms, predicting molecular geometry, and understanding bonding theories. Historically, the concept of bond order was first proposed by Linus Pauling in his seminal work on valence bond theory.
This article will delve into the steps involved in calculating bond order of NO2, exploring its applications and implications in various scientific fields.
How to Calculate Bond Order of NO2
Bond order is a fundamental concept in chemistry that describes the strength and type of chemical bond between atoms. Calculating bond order of NO2 is crucial for understanding the stability and reactivity of this important molecule.
- Molecular Orbital Theory
- Valence Bond Theory
- Resonance Structures
- Hybridization
- Electronegativity
- Bond Length
- Bond Energy
- Magnetic Properties
- Spectroscopic Data
- Thermochemical Data
These aspects are interconnected and provide valuable insights into the electronic structure, bonding, and properties of NO2. Understanding bond order helps chemists predict the behavior and reactivity of molecules, design new materials, and develop novel therapeutic agents.
Molecular Orbital Theory
Molecular Orbital Theory (MO Theory) is a powerful tool for understanding the electronic structure of molecules, including NO2. MO Theory describes the molecular orbitals of a molecule as combinations of atomic orbitals, and it provides a framework for calculating bond order. Bond order is a measure of the strength of a chemical bond, and it is related to the number of electrons in the bonding and antibonding molecular orbitals.
To calculate the bond order of NO2 using MO Theory, we need to first determine the molecular orbitals of NO2. This can be done using various methods, such as the Linear Combination of Atomic Orbitals (LCAO) method. Once the molecular orbitals have been determined, we can calculate the bond order using the following formula:
Bond Order = (Number of electrons in bonding orbitals – Number of electrons in antibonding orbitals) / 2
MO Theory provides a more accurate description of the electronic structure of molecules than Lewis structures, and it can be used to calculate bond orders for a wide variety of molecules. MO Theory is also essential for understanding the bonding in transition metal complexes and organometallic compounds.
Valence Bond Theory
Valence Bond Theory (VB Theory) is a chemical bonding theory that describes the formation of covalent bonds between atoms in terms of overlapping atomic orbitals. VB Theory is closely related to the concept of bond order, which is a measure of the strength of a chemical bond. In the case of NO2, VB Theory can be used to calculate bond order and understand the electronic structure of the molecule.
One of the key postulates of VB Theory is that each covalent bond is formed by the overlap of two atomic orbitals, one from each atom. The extent of overlap determines the strength of the bond, which in turn affects the bond order. In the case of NO2, the two N-O bonds are formed by the overlap of the 2p orbitals on the nitrogen atom with the 2p orbitals on the two oxygen atoms. The overlap between the atomic orbitals is significant, resulting in two strong N-O bonds and a bond order of 2 for each bond.
VB Theory is a powerful tool for understanding the electronic structure and bonding in molecules. It can be used to calculate bond orders, predict molecular geometry, and explain the reactivity of molecules. VB Theory is also used in the design of new materials and drugs.
Resonance Structures
Resonance structures are a fundamental concept in chemistry, helping us comprehend the electronic structure and bonding in molecules like NO2. They provide valuable insights into bond order calculations and offer a deeper understanding of molecular behavior.
- Equivalent Lewis Structures
Resonance structures depict different Lewis structures that represent the same molecular connectivity. For instance, NO2 has two equivalent resonance structures with varying double and single bond placements between nitrogen and oxygen atoms.
- Delocalized Electrons
Resonance structures suggest that certain electrons are not confined to specific bonds but rather spread or ‘delocalized’ across multiple atoms. In NO2, the pi electrons involved in the double bond are delocalized over both N-O bonds, contributing to their equal bond lengths and strengths.
- Bond Order Implications
Resonance structures affect bond order calculations. For NO2, the two resonance structures imply an average bond order of 1.5 for each N-O bond, reflecting the partial double bond character resulting from electron delocalization.
- Chemical Reactivity
Resonance structures provide insights into molecular reactivity. NO2’s resonance structures indicate increased stability due to electron delocalization, influencing its reactions and explaining its role as an important intermediate in atmospheric chemistry.
By considering resonance structures, we gain a more comprehensive understanding of NO2’s electronic structure and bonding. These structures provide a foundation for accurate bond order calculations, helping us unravel the molecular behavior and chemical reactivity of NO2 and other molecules.
Hybridization
In the context of calculating bond order, hybridization plays a crucial role in understanding the electronic structure and bonding in a molecule like NO2. Hybridization involves the combination of atomic orbitals to form new hybrid orbitals with specific shapes and energies, influencing the bond order and molecular geometry.
- Atomic Orbitals
Each atom contributes atomic orbitals, such as s, p, and d orbitals, to the hybridization process.
- Hybrid Orbitals
The atomic orbitals combine to form hybrid orbitals, which have specific shapes and energy levels, such as sp, sp2, and sp3 hybrid orbitals.
- Bond Formation
Hybrid orbitals from different atoms overlap to form sigma () and pi () bonds, determining the bond strength and molecular geometry.
- NO2 Hybridization
In NO2, the nitrogen atom undergoes sp2 hybridization, resulting in three equivalent hybrid orbitals that form sigma bonds with the two oxygen atoms and the unhybridized p orbital on nitrogen forms a pi bond with one oxygen atom.
Understanding hybridization in NO2 helps us determine the bond order, predict the molecular geometry, and explain its reactivity. Hybridization is a fundamental concept in calculating bond order and understanding the electronic structure and bonding in molecules.
Electronegativity
When calculating the bond order of NO2, electronegativity, a measure of an atom’s ability to attract electrons, plays a crucial role in determining the distribution of electrons within the molecule and the strength of the chemical bonds.
- Electronegativity Difference
Electronegativity difference between atoms influences bond polarity and bond order. In NO2, the greater electronegativity of oxygen compared to nitrogen results in a polar covalent bond, affecting bond order calculations.
- Electronegativity and Resonance
Electronegativity affects the resonance structures of NO2. The ability of oxygen to withdraw electrons through resonance stabilizes the molecule, influencing bond order determination.
- Hybridization and Electronegativity
Electronegativity influences the hybridization of the nitrogen atom in NO2. The sp2 hybridization of nitrogen is influenced by the electronegativity of oxygen, impacting bond angles and bond order.
Understanding electronegativity and its effects on bond order in NO2 is essential for accurately predicting molecular properties, reactivity, and behavior. Electronegativity provides valuable insights into the electronic structure and bonding characteristics of NO2, aiding in the comprehension of its chemical nature.
Bond Length
In the context of calculating bond order in NO2, bond length plays a critical role in determining the strength and nature of the chemical bond between nitrogen and oxygen atoms. Bond length is inversely related to bond order, meaning that shorter bond lengths correspond to higher bond orders and vice versa.
The bond order of NO2 can be calculated using various methods, including molecular orbital theory and valence bond theory. These methods take into account the number and type of atomic orbitals involved in the bond, as well as the electronegativity of the atoms. Bond length is a key experimental parameter that can be used to validate the calculated bond order.
For instance, in NO2, the bond length between the nitrogen and oxygen atoms is approximately 1.19 angstroms. This relatively short bond length indicates a strong bond with a bond order of 2, which is consistent with the results obtained from theoretical calculations. Understanding the relationship between bond length and bond order is crucial for accurately predicting the properties and reactivity of molecules.
Bond Energy
Bond energy is a crucial aspect of understanding and calculating bond order in NO2. It represents the amount of energy required to break a chemical bond between two atoms. Bond energy is directly related to bond order, with stronger bonds having higher bond orders and higher bond energies. In the case of NO2, the bond energy of the N-O bond is approximately 200 kJ/mol. This relatively high bond energy indicates a strong bond with a bond order of 2, which is consistent with the results obtained from theoretical calculations.
Understanding the relationship between bond energy and bond order is essential for accurately predicting the properties and reactivity of molecules. For instance, in NO2, the high bond energy of the N-O bond contributes to the stability of the molecule and its resistance to dissociation. This understanding is critical in various fields, such as atmospheric chemistry, where NO2 plays a role in ozone depletion.
In summary, bond energy and bond order are closely interconnected concepts that provide valuable insights into the electronic structure and bonding characteristics of molecules. By considering both bond energy and bond order, scientists can gain a deeper understanding of molecular properties, reactivity, and behavior.
Magnetic Properties
Magnetic properties play a significant role in the calculation of bond order in NO2, offering valuable insights into the electronic structure and bonding characteristics of the molecule.
- Magnetic Susceptibility
Magnetic susceptibility measures the degree to which a substance can be magnetized. For NO2, its magnetic susceptibility helps determine the number of unpaired electrons, which influences the bond order calculations.
- Electron Paramagnetic Resonance (EPR)
EPR spectroscopy provides information about unpaired electrons in NO2. By studying the EPR spectrum, researchers can gain insights into the electronic structure and bonding, aiding in bond order determination.
- Nuclear Magnetic Resonance (NMR)
NMR spectroscopy helps determine the chemical environment and connectivity of atoms in NO2. Analyzing the NMR spectrum provides information about the electron density distribution and bond order.
- Magnetism and Reactivity
The magnetic properties of NO2 influence its reactivity. For instance, the presence of unpaired electrons can affect the molecule’s susceptibility to certain chemical reactions, impacting its behavior in various chemical processes.
In summary, magnetic properties offer a multifaceted approach to understanding bond order in NO2. By investigating magnetic susceptibility, EPR, NMR, and the relationship between magnetism and reactivity, scientists can gain a deeper understanding of the electronic structure, bonding characteristics, and chemical behavior of NO2.
Spectroscopic Data
Spectroscopic data plays a crucial role in calculating bond order of NO2, providing valuable insights into the molecule’s electronic structure and bonding characteristics. Various spectroscopic techniques offer complementary information, contributing to a comprehensive understanding of NO2’s bond order.
- UV-Visible Spectroscopy
UV-Visible spectroscopy measures the absorption of light in the ultraviolet and visible regions. By analyzing the absorption spectrum of NO2, researchers can determine the energy difference between molecular orbitals, aiding in bond order calculations.
- Infrared Spectroscopy
Infrared spectroscopy analyzes the absorption of infrared radiation. The vibrational frequencies observed in the infrared spectrum provide information about bond strengths and types, contributing to the determination of bond order in NO2.
- Raman Spectroscopy
Raman spectroscopy involves the inelastic scattering of light. The Raman spectrum provides insights into molecular vibrations and bond strengths, complementing the data obtained from infrared spectroscopy for bond order calculations.
- Electron Spin Resonance (ESR) Spectroscopy
ESR spectroscopy detects and characterizes unpaired electrons in molecules. For NO2, ESR spectroscopy can provide information about the presence and number of unpaired electrons, influencing the calculation of bond order.
By combining data from various spectroscopic techniques, scientists can gain a comprehensive understanding of the electronic structure and bonding in NO2, enabling accurate bond order calculations. These insights are essential for predicting molecular properties, reactivity, and behavior in chemical processes.
Thermochemical Data
Thermochemical data plays a crucial role in calculating bond order of NO2, providing valuable insights into the molecule’s energetic properties and bonding characteristics.
- Enthalpy Change (H)
Enthalpy change measures the heat absorbed or released during a chemical reaction. For NO2 formation, H provides information about the energy required to break and form bonds, contributing to the calculation of bond order.
- Entropy Change (S)
Entropy change measures the change in disorder or randomness during a reaction. S data helps determine the stability and spontaneity of NO2 formation, influencing bond order calculations.
- Gibbs Free Energy Change (G)
Gibbs free energy change combines enthalpy and entropy changes to predict the spontaneity and feasibility of a reaction under specific conditions. G data aids in understanding the stability and reactivity of NO2, impacting bond order calculations.
- Heat Capacity (Cp)
Heat capacity measures the amount of heat required to raise the temperature of a substance. Cp data provides insights into the vibrational and rotational motions of NO2 molecules, contributing to the calculation of bond strength and bond order.
Thermochemical data offers a comprehensive view of the energetics and properties of NO2, enabling accurate bond order calculations. By incorporating these data into theoretical models, scientists can gain a deeper understanding of NO2’s behavior and reactivity in various chemical processes.
FAQs on Calculating Bond Order of NO2
This section addresses frequently asked questions and clarifies key aspects of calculating bond order in NO2.
Question 1: What is bond order and why is it important?
Bond order describes the strength and type of chemical bond between atoms. It helps predict molecular properties, reactivity, and stability.
Question 2: How do I calculate bond order using molecular orbital theory?
Use the formula: Bond Order = (Number of electrons in bonding orbitals – Number of electrons in antibonding orbitals) / 2.
Question 3: How does resonance affect bond order in NO2?
Resonance structures indicate delocalized electrons, affecting bond order. In NO2, resonance leads to an average bond order of 1.5 for each N-O bond.
Question 4: What is the relationship between bond order and bond length?
Bond order and bond length are inversely related. Shorter bond lengths indicate higher bond orders and vice versa.
Question 5: How can I use spectroscopic data to determine bond order?
Spectroscopic techniques, such as UV-Visible and infrared spectroscopy, provide information about molecular orbitals and bond strengths, aiding in bond order calculations.
Question 6: What are some applications of calculating bond order?
Bond order calculations are used in drug design, material science, and understanding chemical reactions and molecular properties.
These FAQs provide essential insights into calculating bond order of NO2, laying the groundwork for further exploration of its applications and implications.
Next Section: Advanced Techniques for Bond Order Calculations
Tips for Calculating Bond Order of NO2
This section presents practical tips to enhance your understanding and accuracy when calculating bond order of NO2.
Tip 1: Master Basic Concepts
Grasp fundamental principles like atomic orbitals, hybridization, and molecular orbital theory to lay a solid foundation.
Tip 2: Utilize Multiple Methods
Employ various approaches, such as molecular orbital theory and valence bond theory, to cross-validate your results and gain a comprehensive perspective.
Tip 3: Consider Resonance Structures
Account for resonance to determine the average bond order and understand electron delocalization in NO2.
Tip 4: Examine Bond Properties
Analyze bond length, bond energy, and magnetic properties to corroborate your bond order calculations.
Tip 5: Leverage Spectroscopic Data
Utilize spectroscopic techniques (e.g., UV-Visible, IR) to gather experimental evidence supporting your bond order determinations.
Tip 6: Apply Computational Tools
Employ computational chemistry software to perform advanced calculations and visualize molecular orbitals.
Tip 7: Consult Reference Materials
Refer to textbooks, research papers, and online resources for additional insights and examples.
Tip 8: Practice and Validate
Regularly practice bond order calculations and compare your results with established values to refine your skills.
By implementing these tips, you will enhance the accuracy and depth of your bond order calculations, gaining a stronger grasp of NO2’s electronic structure and chemical properties.
Next Section: Advanced Techniques for Bond Order Calculations
Conclusion
This article has provided a comprehensive overview of how to calculate bond order of NO2, exploring various theoretical and experimental approaches. We have highlighted the significance of molecular orbital theory, valence bond theory, and resonance structures in determining bond order, emphasizing the interplay between these concepts.
Furthermore, we have discussed the importance of considering bond properties such as bond length, bond energy, and magnetic properties to corroborate bond order calculations. The utilization of spectroscopic data and computational tools has also been emphasized as valuable techniques for gaining insights into the electronic structure and bonding characteristics of NO2.
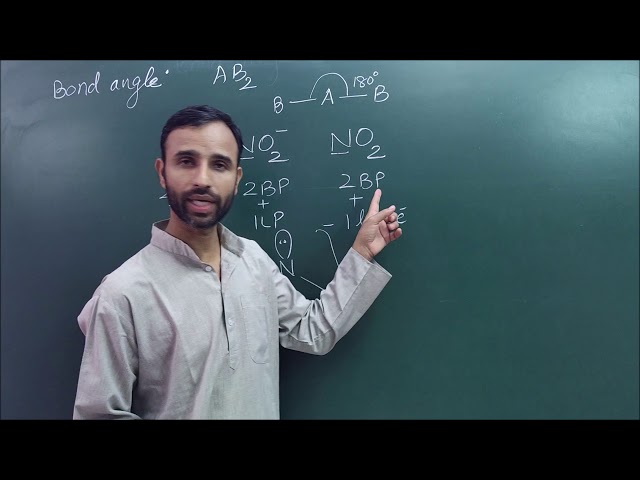