Calculating bond order of O3 involves determining the number of bonds between atoms in an O3 molecule. This concept is fundamental in chemistry as it helps comprehend the structure and properties of molecules.
Bond order calculations provide insights into molecular stability, reactivity, and physical characteristics. Understanding bond order has aided in designing materials with specific properties, such as in the field of nanotechnology. Historically, the concept of bond order was first proposed by Linus Pauling in 1931.
This article will delve into the detailed steps of calculating bond order in O3, exploring the molecular orbital theory and resonance structures involved in this process.
How to Calculate Bond Order of O3
Understanding the essential aspects of calculating bond order in O3 is crucial for comprehending its molecular structure and properties.
- Molecular Orbital Theory
- Resonance Structures
- Bond Length
- Bond Energy
- Molecular Stability
- Reactivity
- Physical Properties
- Nanotechnology Applications
- Historical Developments
These aspects encompass the theoretical foundations, experimental observations, and practical applications related to bond order calculations in O3. By examining these aspects, we gain a deeper understanding of the behavior and significance of O3 at the molecular level.
Molecular Orbital Theory
Molecular Orbital Theory (MOT) provides a conceptual framework for understanding chemical bonding in O3, allowing us to calculate bond order and predict molecular properties.
- Atomic Orbitals: Electrons in O3 occupy specific atomic orbitals, which are mathematical functions describing their wave-like behavior.
- Linear Combination of Atomic Orbitals (LCAO): MOT combines atomic orbitals to form molecular orbitals, which extend over the entire molecule and describe the bonding interactions between atoms.
- Molecular Orbital Energy Levels: The energy levels of molecular orbitals determine the stability and reactivity of the molecule.
- Bonding and Antibonding Orbitals: MOT identifies bonding orbitals, which promote electron density between atoms, and antibonding orbitals, which reduce electron density and weaken bonds.
These facets of MOT collectively contribute to calculating bond order in O3 by providing a detailed description of electron distribution and bonding interactions within the molecule.
Resonance Structures
Resonance structures are crucial for accurately calculating bond order in O3. They depict the delocalization of electrons within the molecule, providing a more comprehensive view of its electronic structure. This delocalization influences the bond order and, consequently, the overall properties of O3.
In O3, resonance structures arise due to the presence of multiple resonance forms. These forms result from the movement of electrons within the molecule, leading to alternating single and double bonds between the oxygen atoms. The resonance structures contribute to the stability of O3 by distributing the electron density more evenly.
To calculate bond order in O3, one must consider the contributions from all resonance structures. The bond order is then calculated as the average number of bonds between the atoms involved, taking into account the resonance structures’ relative importance. This approach provides a more accurate representation of the bonding in O3 compared to using a single Lewis structure.
Understanding resonance structures is essential for various applications involving O3. For instance, in atmospheric chemistry, O3 plays a vital role in protecting the Earth from harmful ultraviolet radiation. Accurate calculations of its bond order help determine its stability and reactivity in the atmosphere.
Bond Length
Bond Length is a critical aspect in calculating bond order of O3, providing valuable insights into the molecule’s structure and properties.
- Atomic Radii: The atomic radii of oxygen atoms determine the minimum distance between their nuclei, influencing the bond length in O3.
- Bond Order: Bond order is inversely proportional to bond length, meaning stronger bonds (higher bond order) are shorter.
- Electronegativity: The electronegativity difference between oxygen atoms affects bond length, with more electronegative atoms pulling electron density towards themselves and shortening the bond.
- Resonance: Resonance in O3 leads to delocalization of electrons, which can affect bond lengths by distributing electron density more evenly and altering bond strengths.
Understanding Bond Length allows chemists to predict molecular geometry, stability, and reactivity. For example, the shorter bond lengths in O3 contribute to its higher reactivity compared to O2, making it an effective oxidizing agent in atmospheric chemistry.
Bond Energy
Bond Energy plays a significant role in determining the bond order of O3. It represents the energy required to break a bond between two atoms and provides insights into the strength and stability of the molecule.
- Bond Dissociation Energy: The energy needed to break a specific bond in a molecule, providing a direct measure of bond strength.
- Bond Order: Bond energy is directly proportional to bond order, meaning stronger bonds (higher bond order) have higher bond energies.
- Resonance: Resonance in O3 leads to delocalization of electrons, which can affect bond energies by distributing electron density more evenly and altering bond strengths.
- Molecular Stability: Bond energies contribute to the overall stability of O3, influencing its reactivity and behavior in various chemical reactions.
Understanding Bond Energy allows chemists to predict the reactivity and stability of O3 in different environments. For instance, the high bond energies in O3 make it a relatively unreactive molecule, contributing to its persistence in the Earth’s atmosphere as the protective ozone layer.
Molecular Stability
Molecular stability is a crucial aspect in determining the bond order of O3. Bond order, which represents the strength and multiplicity of chemical bonds between atoms, directly influences the stability of the molecule. A higher bond order generally corresponds to a more stable molecule.
In the case of O3, the bond order can be calculated using molecular orbital theory, which considers the number and type of molecular orbitals involved in bonding. A higher bond order indicates a greater number of bonding orbitals and fewer antibonding orbitals, resulting in a more stable O3 molecule.
Understanding the relationship between bond order and molecular stability has practical applications in various fields. For instance, in atmospheric chemistry, O3 plays a vital role in protecting the Earth from harmful ultraviolet radiation. Accurate calculations of its bond order help determine its stability and reactivity in the atmosphere, which is crucial for predicting its effectiveness as a protective layer.
Reactivity
In the context of calculating bond order of O3, “Reactivity” refers to the molecule’s propensity to undergo chemical reactions and interact with other substances. Understanding reactivity provides valuable insights into the behavior and applications of O3 in various fields.
- Reaction Rates: Bond order influences the rate at which O3 reacts with other molecules. Higher bond order generally leads to lower reactivity, as stronger bonds require more energy to break.
- Oxidizing Ability: O3 is a potent oxidizing agent, and its bond order affects its oxidizing strength. Higher bond order corresponds to weaker oxidizing ability, as the molecule becomes more stable and less likely to donate electrons.
- Atmospheric Chemistry: In the Earth’s atmosphere, O3 plays a crucial role in absorbing ultraviolet radiation. Its bond order determines its stability and reactivity in this environment, influencing its effectiveness as a protective layer.
- Industrial Applications: O3 is used in various industrial processes, such as water purification and air pollution control. Calculating its bond order helps predict its reactivity and optimize its use in these applications.
By understanding the reactivity of O3 through the lens of bond order, scientists and researchers can gain deeper insights into its behavior and tailor its applications in diverse fields, contributing to advancements in atmospheric science, industrial chemistry, and environmental protection.
Physical Properties
In understanding how to calculate bond order of O3, it is essential to consider its physical properties. These properties offer valuable insights into the molecule’s behavior and characteristics.
- Molecular Geometry: The bond order of O3 influences its molecular geometry, determining the arrangement of atoms in space and affecting its reactivity.
- Bond Lengths: The bond order is inversely proportional to bond lengths, meaning stronger bonds (higher bond order) are shorter.
- Magnetic Properties: The bond order affects the magnetic properties of O3, as it determines the number of unpaired electrons in the molecule.
- Electrical Conductivity: The bond order can influence the electrical conductivity of O3, with higher bond order generally leading to lower conductivity.
Understanding these physical properties provides a comprehensive view of O3‘s structure and behavior. By considering bond order in conjunction with physical properties, scientists can gain a deeper understanding of this molecule and its applications in various fields.
Nanotechnology Applications
The field of nanotechnology involves manipulating and utilizing materials at the nanoscale, ranging from 1 to 100 nanometers in size. Understanding how to calculate bond order of O3 plays a critical role in the design and development of various nanotechnology applications.
In nanotechnology, controlling the structure and properties of materials at the atomic and molecular level is essential. The bond order of O3, which represents the strength and nature of the chemical bonds between oxygen atoms, directly influences these properties. By precisely calculating and manipulating the bond order, scientists can engineer materials with tailored electronic, optical, and mechanical properties for specific applications.
One prominent example of a nanotechnology application where bond order calculations are crucial is in the development of nano-catalysts. Catalysts are substances that enhance the rate of chemical reactions without being consumed themselves. By optimizing the bond order of O3 in nano-catalysts, researchers can improve their catalytic activity and selectivity, leading to more efficient and environmentally friendly industrial processes.
In summary, understanding how to calculate bond order of O3 is a fundamental aspect of nanotechnology applications. It enables scientists to design and engineer materials with precise properties, opening up new possibilities for advancements in diverse fields such as energy storage, electronics, and biomedical engineering.
Historical Developments
Historical developments in chemistry have significantly shaped our understanding of how to calculate bond order of O3. The concept of bond order was first proposed by Linus Pauling in 1931, providing a groundbreaking framework for describing the strength and nature of chemical bonds.
Pauling’s theory was based on quantum mechanics and the molecular orbital theory, which revolutionized our understanding of atomic and molecular structures. By considering the wave-like behavior of electrons, Pauling developed a method to calculate bond order based on the number and type of molecular orbitals involved in bonding. This approach laid the foundation for modern methods used to calculate bond order in O3 and other molecules.
Over the years, advancements in computational chemistry and experimental techniques have further refined our ability to calculate bond order with greater accuracy and efficiency. These developments have enabled scientists to gain deeper insights into the electronic structure and properties of O3, leading to a better understanding of its behavior in various chemical reactions and applications.
In summary, historical developments in chemistry, particularly the introduction of molecular orbital theory and the contributions of Linus Pauling, have been instrumental in establishing the theoretical basis and practical methods for calculating bond order of O3. This understanding is crucial for predicting molecular properties, reactivity, and behavior, which has broad applications in fields such as atmospheric chemistry, materials science, and nanotechnology.
Frequently Asked Questions about Calculating Bond Order of O3
This section provides answers to frequently asked questions about calculating bond order of O3, addressing common concerns and clarifying key aspects of this concept.
Question 1: What is bond order, and why is it important?
Answer: Bond order describes the strength and multiplicity of chemical bonds between atoms. It helps predict molecular stability, reactivity, and various physical properties.
Question 2: How do I calculate the bond order of O3?
Answer: To calculate the bond order of O3, you can use molecular orbital theory or resonance structures to determine the number and type of bonds between the oxygen atoms.
Question 3: What factors influence the bond order of O3?
Answer: Factors affecting bond order in O3 include the electronegativity of oxygen atoms, the presence of resonance structures, and the overall molecular orbital interactions.
Question 4: How does bond order relate to the stability of O3?
Answer: Bond order is directly proportional to molecular stability. Higher bond order indicates stronger bonds and a more stable O3 molecule.
Question 5: What are the applications of calculating bond order in O3?
Answer: Understanding bond order in O3 is crucial for predicting its reactivity in atmospheric chemistry, designing nano-catalysts, and engineering materials with specific properties.
Question 6: What are the limitations of calculating bond order?
Answer: Bond order calculations provide an approximation of bond strength and do not always accurately reflect the complex electronic interactions in molecules.
These FAQs provide essential insights into the concept of bond order in O3, its calculation methods, and its applications. Understanding these aspects is crucial for further exploring the behavior and properties of O3 in various chemical and scientific contexts.
In the next section, we will delve deeper into the experimental techniques and computational methods used to calculate bond order in O3, providing a comprehensive overview of this important concept.
Tips for Calculating Bond Order of O3
To enhance your understanding and accuracy when calculating bond order in O3, consider implementing these practical tips:
Tip 1: Utilize molecular orbital theory to determine the number and type of molecular orbitals involved in bonding.Tip 2: Consider resonance structures to account for electron delocalization and its effects on bond order.Tip 3: Employ computational chemistry software to perform complex calculations and visualize molecular orbitals.Tip 4: Understand the relationship between bond order, bond length, and bond energy to gain insights into molecular stability.Tip 5: Consider the electronegativity of atoms and the hybridization of orbitals when analyzing bond order.Tip 6: Validate your calculations using experimental techniques such as X-ray crystallography or spectroscopy.Tip 7: Consult reputable literature and scientific databases for additional information and examples.Tip 8: Practice regularly to improve your skills and confidence in bond order calculations.
By following these tips, you can refine your approach, obtain more accurate results, and deepen your understanding of bond order in O3 and other molecules.
These practical tips lay the groundwork for the concluding section, where we will explore advanced applications and future directions in bond order calculations.
Conclusion
This comprehensive exploration of bond order calculation in O3 provided a deep dive into the theoretical foundations, experimental techniques, and practical applications of this concept. We examined the role of molecular orbital theory, resonance structures, and computational methods in determining bond order, gaining valuable insights into molecular stability, reactivity, and physical properties.
Key takeaways include the direct relationship between bond order and molecular stability, the influence of resonance on bond order distribution, and the significance of bond order in understanding O3‘s behavior in atmospheric chemistry and nanotechnology. By unraveling these intricate connections, we not only enhance our comprehension of O3 but also pave the way for future advancements in diverse scientific fields.
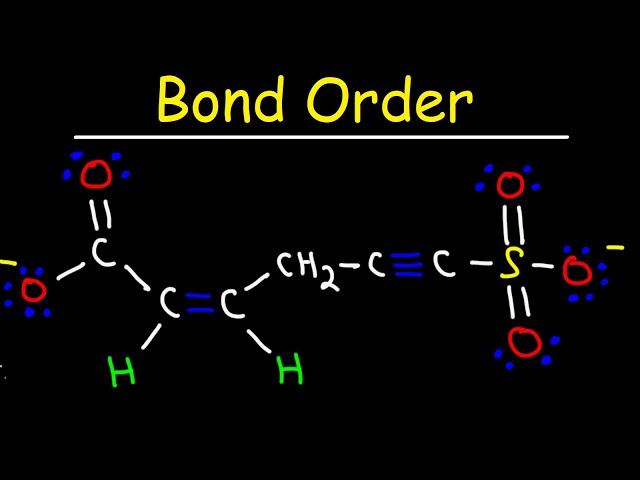