Calculating the bond order of sulfate ion (SO42-) provides valuable insights into its molecular structure and chemical properties. Bond order refers to the number of chemical bonds between two atoms in a molecule. A higher bond order indicates a stronger and more stable bond.
Bond order is a crucial concept in chemistry, as it influences various molecular properties, including bond length, strength, and reactivity. Understanding bond order is essential for predicting the behavior and stability of molecules. One notable historical development in this field was Linus Pauling’s introduction of the concept of resonance, which expanded the understanding of bond order in molecules with multiple resonance structures.
This article will delve into the step-by-step process of calculating the bond order of sulfate ion and explore its significance in understanding the molecular structure and properties of this important compound.
How to Calculate Bond Order of SO42-
Understanding the essential aspects of calculating bond order in sulfate ion (SO42-) is crucial for comprehending its molecular structure and properties.
- Resonance Structures
- Formal Charges
- Molecular Orbitals
- Hybrid Orbitals
- Electron Delocalization
- Bond Length
- Bond Strength
- Molecular Geometry
- Spectroscopic Properties
- Chemical Reactivity
These aspects are interconnected and provide a comprehensive understanding of SO42-‘s bonding and behavior. For instance, resonance structures help visualize the electron delocalization within the ion, influencing bond lengths and strengths. Molecular orbitals describe the distribution of electrons in the ion, providing insights into its electronic structure and reactivity. Understanding these aspects enables chemists to predict and explain the properties and reactions of sulfate ion in various chemical systems.
Resonance Structures
Resonance structures are crucial for understanding the bonding and properties of sulfate ion (SO42-). They provide a way to represent the delocalization of electrons within the ion, which affects the bond order and other molecular properties.
- Equivalent Resonance Forms: SO42- has two equivalent resonance forms, which means that the electrons are evenly distributed between the four oxygen atoms. This delocalization results in the formation of four equal S-O bonds, each with a bond order of 1.5.
- Lewis Dot Structures: The resonance structures of SO42- can be drawn using Lewis dot structures. Each oxygen atom has six valence electrons, and the sulfur atom has six valence electrons. The electrons are arranged so that each atom has a complete octet of electrons.
- Formal Charges: The formal charge on each atom in the resonance structures of SO42- is zero. This means that the electrons are evenly distributed among the atoms, and there is no net charge on the ion.
- Molecular Geometry: The resonance structures of SO42- help to explain the ion’s molecular geometry. The ion has a tetrahedral shape, with the sulfur atom at the center and the four oxygen atoms arranged around it. The bond angles are all approximately 109.5 degrees.
Overall, resonance structures are a powerful tool for understanding the bonding and properties of sulfate ion. They provide a way to visualize the delocalization of electrons within the ion and to explain the ion’s molecular geometry and other properties.
Formal Charges
In the context of calculating bond order, formal charges play a crucial role in determining the distribution of electrons within a molecule or ion. Formal charges are hypothetical charges assigned to each atom in a molecule, assuming that electrons are localized and that each atom “owns” the electrons it shares with its bonded neighbors. These charges provide insights into the polarity of bonds and the stability of molecular structures.
When calculating the bond order of sulfate ion (SO42-), formal charges are used to determine the resonance structures and the electron delocalization within the ion. By assigning formal charges to each atom, chemists can identify the most stable resonance structure, which contributes to the overall bond order of the ion. The formal charges on the sulfur and oxygen atoms in SO42- are zero, indicating that the electrons are evenly distributed among the atoms.
Understanding formal charges is essential for accurately calculating bond order, as it helps determine the resonance structures and the electron distribution within the molecule or ion. This understanding enables chemists to predict and explain the properties and reactivity of various chemical species, including sulfate ion. Formal charges provide valuable insights into the electronic structure and bonding of molecules, facilitating a deeper comprehension of their chemical behavior.
Molecular Orbitals
Molecular orbitals play a crucial role in calculating the bond order of sulfate ion (SO42-). Molecular orbitals are mathematical functions that describe the wave-like behavior of electrons within a molecule or ion. They provide insights into the distribution and energy of electrons, which is essential for understanding the bonding and properties of chemical species.
In the case of SO42-, molecular orbitals are used to determine the resonance structures and the delocalization of electrons within the ion. By constructing molecular orbitals and analyzing their interactions, chemists can determine the most stable resonance structure, which contributes to the overall bond order of the ion. The molecular orbitals of SO42- consist of bonding, antibonding, and non-bonding orbitals. The bonding orbitals are formed by the overlap of atomic orbitals on adjacent atoms, resulting in electron density concentration between the nuclei and the formation of chemical bonds. The antibonding orbitals, on the other hand, result from the out-of-phase combination of atomic orbitals, leading to electron density depletion between the nuclei and weakening of bonds.
Understanding molecular orbitals is critical for accurately calculating bond order, as it provides insights into the electronic structure and bonding of the molecule or ion. This understanding enables chemists to predict and explain the properties and reactivity of various chemical species, including SO42-. Molecular orbitals have practical applications in various fields, such as quantum chemistry, materials science, and drug design. By manipulating molecular orbitals, scientists can design materials with specific properties or develop drugs that target specific biological molecules.
Hybrid Orbitals
Hybrid orbitals play a pivotal role in understanding and calculating the bond order of sulfate ion (SO42-). Hybrid orbitals are formed by the mixing of atomic orbitals, resulting in new orbitals with specific shapes and energies. In the case of SO42-, the sulfur atom undergoes hybridization, leading to the formation of four equivalent sp3 hybrid orbitals.
These sp3 hybrid orbitals overlap with the p-orbitals of the four oxygen atoms, forming four S-O sigma bonds. The tetrahedral arrangement of the sp3 hybrid orbitals results in the tetrahedral molecular geometry of SO42-. The bond order of each S-O bond can be calculated by determining the number of electron pairs shared between the sulfur and oxygen atoms and the extent of overlap between their respective orbitals.
Understanding hybrid orbitals is crucial for accurately calculating the bond order of SO42-, as it provides insights into the electronic structure and bonding of the ion. This understanding enables chemists to predict and explain the properties and reactivity of sulfate ion in various chemical systems. Hybrid orbitals have practical applications in diverse fields such as inorganic chemistry, materials science, and biochemistry. By manipulating hybrid orbitals, scientists can design materials with specific properties or develop drugs that target specific biological molecules.
Electron Delocalization
Electron delocalization plays a crucial role in understanding and calculating the bond order of sulfate ion (SO42-). It involves the spreading or distribution of electrons over multiple atoms or regions within a molecule or ion, resulting in a more stable and uniform electron distribution.
- Resonance Structures: Electron delocalization in SO42- is evident in its resonance structures. The two equivalent resonance structures indicate that the electrons are delocalized over the four oxygen atoms, leading to an equal distribution of electron density and the formation of four equivalent S-O bonds.
- Molecular Orbitals: Molecular orbital theory further explains electron delocalization in SO42-. The bonding molecular orbitals resulting from the overlap of atomic orbitals are spread over the entire molecule, contributing to the delocalization of electrons and the stability of the ion.
- Hybrid Orbitals: The hybridization of the sulfur atom’s orbitals also contributes to electron delocalization. The sp3 hybrid orbitals overlap with the p-orbitals of the oxygen atoms, resulting in the formation of four equivalent sigma bonds and the tetrahedral molecular geometry of SO42-.
- Bond Order: The delocalization of electrons in SO42- affects the bond order. The bond order, which represents the number of chemical bonds between atoms, is determined by the number of electron pairs shared between them. In SO42-, the electron delocalization results in a bond order of 1.5 for each S-O bond.
Understanding electron delocalization is essential for accurately calculating the bond order of SO42- and for comprehending the electronic structure and bonding of the ion. This understanding enables chemists to predict and explain the properties and reactivity of sulfate ion in various chemical systems.
Bond Length
In the context of calculating the bond order of sulfate ion (SO42-), bond length plays a crucial role in determining the strength and nature of the chemical bonds within the ion. Bond length refers to the distance between the nuclei of two bonded atoms and provides valuable insights into the electronic structure and properties of the molecule or ion.
- Atomic Radii: The bond length between two atoms is influenced by their atomic radii. Atoms with larger atomic radii tend to form longer bonds, while atoms with smaller atomic radii tend to form shorter bonds.
- Bond Order: Bond length is inversely proportional to bond order. Higher bond orders indicate shorter bond lengths due to increased electron density and stronger bonding interactions between the atoms.
- Hybridization: The hybridization of atomic orbitals affects bond length. Hybrid orbitals with more p-character result in shorter bond lengths because p-orbitals overlap more effectively, leading to stronger bonds.
- Electronegativity: The electronegativity of the bonded atoms influences bond length. Atoms with higher electronegativity tend to attract electrons towards themselves, resulting in shorter bond lengths due to increased electrostatic attraction.
Understanding bond length is essential for accurately calculating the bond order of SO42- and for comprehending the electronic structure and bonding of the ion. By considering the factors that influence bond length, chemists can gain insights into the properties and reactivity of sulfate ion in various chemical systems.
Bond Strength
In the context of calculating the bond order of sulfate ion (SO42-), bond strength holds significant importance as it directly influences the stability and properties of the ion. Bond strength refers to the force that holds atoms together in a chemical bond and is primarily determined by the number and type of bonds between the atoms.
Bond strength and bond order are closely related. Higher bond orders indicate stronger bonds, as they represent a greater number of electron pairs shared between the bonded atoms. In the case of SO42-, the bond order of each S-O bond is 1.5, indicating that there is a strong covalent bond between the sulfur and oxygen atoms. This strong bond strength contributes to the stability and overall structure of the ion.
Understanding bond strength is crucial for accurately calculating the bond order of SO42- and for comprehending the electronic structure and bonding of the ion. By considering the factors that influence bond strength, such as bond order, electronegativity, and atomic radii, chemists can gain insights into the properties and reactivity of sulfate ion in various chemical systems. This understanding has practical applications in diverse fields such as inorganic chemistry, materials science, and biochemistry.
Molecular Geometry
Molecular geometry, the three-dimensional arrangement of atoms within a molecule, plays a crucial role in determining the bond order of sulfate ion (SO42-). The molecular geometry of SO42- is tetrahedral, with the sulfur atom at the center and the four oxygen atoms arranged at the corners of a tetrahedron. This tetrahedral geometry is a direct consequence of the ion’s electronic structure and the hybridization of the sulfur atom’s orbitals.
The bond order of a chemical bond is a measure of its strength and is determined by the number of electron pairs shared between the bonded atoms. In the case of SO42-, each sulfur-oxygen bond has a bond order of 1.5. This bond order is consistent with the tetrahedral molecular geometry of the ion, as the four sp3 hybrid orbitals of the sulfur atom overlap with the p-orbitals of the four oxygen atoms to form four equivalent sigma bonds. The tetrahedral geometry maximizes the overlap between these orbitals, resulting in strong and stable bonds.
Understanding the relationship between molecular geometry and bond order is essential for accurately calculating the bond order of SO42- and for comprehending the electronic structure and bonding of the ion. This understanding has practical applications in diverse fields such as inorganic chemistry, materials science, and biochemistry. For example, in the design of new materials, controlling the molecular geometry of a compound can influence its physical and chemical properties, such as its strength, conductivity, and reactivity. Similarly, in biochemistry, understanding the molecular geometry of biological molecules is crucial for understanding their function and interactions within living organisms.
Spectroscopic Properties
Spectroscopic properties play a crucial role in understanding and calculating the bond order of sulfate ion (SO42-). Spectroscopy is the study of the interaction of light with matter, and it provides valuable insights into the electronic structure and bonding of molecules and ions. In the case of SO42-, spectroscopic techniques such as infrared (IR) and Raman spectroscopy can be used to probe the vibrational modes of the ion, which are sensitive to the bond order and strength.
The vibrational modes of a molecule or ion correspond to the different ways in which its atoms can vibrate. The frequency of a vibrational mode is determined by the strength of the bond and the masses of the atoms involved. In SO42-, the S-O stretching vibration is a particularly important mode for understanding the bond order. The frequency of this vibration is inversely proportional to the bond order, meaning that a higher bond order will result in a higher vibrational frequency. By measuring the vibrational frequency of the S-O stretching mode using IR or Raman spectroscopy, it is possible to determine the bond order of SO42-.
The understanding of the relationship between spectroscopic properties and bond order has practical applications in various fields. For example, in inorganic chemistry, it is used to characterize and identify different sulfate-containing compounds. In materials science, it is used to study the structure and properties of sulfate-based materials. Additionally, in biochemistry, it is used to investigate the role of sulfate ions in biological systems.
Chemical Reactivity
Chemical reactivity is a crucial aspect of understanding the behavior and properties of sulfate ion (SO42-) and its role in various chemical reactions. By examining the chemical reactivity of SO42-, we can gain insights into its stability, reactivity patterns, and potential applications.
- Nucleophilic Substitution:
SO42- can act as a nucleophile, attacking electrophilic centers in other molecules to form new bonds. This reactivity is important in many organic and inorganic reactions, such as the hydrolysis of esters and the formation of sulfate salts.
- Redox Reactions:
SO42- can participate in redox reactions, undergoing oxidation or reduction depending on the reaction conditions. Understanding the redox behavior of SO42- is essential in fields such as electrochemistry and environmental chemistry.
- Acid-Base Reactions:
SO42- is a weak base and can undergo acid-base reactions with strong acids to form hydrogen sulfate ions (HSO4–). This reactivity is important in many industrial processes, such as the production of fertilizers and the treatment of wastewater.
- Complex Formation:
SO42- can form complexes with metal ions to create coordination compounds. The stability and properties of these complexes depend on the bond order of the S-O bonds in SO42-, highlighting the importance of bond order calculations in understanding complex formation.
By understanding the chemical reactivity of SO42- and its relationship to bond order, chemists can predict and control the behavior of this important ion in a wide range of applications, from industrial processes to environmental remediation. The insights gained from bond order calculations provide a valuable foundation for exploring the multifaceted chemical reactivity of sulfate ion.
Frequently Asked Questions about Calculating Bond Order of SO42-
This section addresses common questions and clarifies key aspects related to calculating the bond order of sulfate ion (SO42-).
Question 1: What is bond order and why is it important for SO42-?
Bond order describes the number of chemical bonds between two atoms. For SO42-, understanding bond order helps determine its molecular structure, stability, and chemical reactivity.
Question 2: How do I calculate the bond order of SO42-?
To calculate the bond order, you need to consider resonance structures, formal charges, molecular orbitals, and hybridization, which provide insights into the electron distribution and bonding within the ion.
Question 3: What is the relationship between bond order and bond length?
Bond order is inversely proportional to bond length. Higher bond order indicates shorter bond lengths due to increased electron density and stronger bonding interactions.
Question 4: How does bond order affect the molecular geometry of SO42-?
The bond order of SO42- determines its tetrahedral molecular geometry. The four equivalent S-O bonds, each with a bond order of 1.5, result in a tetrahedral arrangement of the oxygen atoms around the central sulfur atom.
Question 5: What experimental techniques can be used to determine the bond order of SO42-?
Spectroscopic techniques, such as infrared (IR) and Raman spectroscopy, can be used to probe the vibrational modes of SO42-. The frequency of the S-O stretching vibration is inversely proportional to the bond order, providing an experimental method for its determination.
Question 6: How does bond order influence the chemical reactivity of SO42-?
The bond order of SO42- affects its reactivity in nucleophilic substitution, redox reactions, acid-base reactions, and complex formation. Understanding bond order is crucial for predicting and controlling the chemical behavior of sulfate ion in various applications.
These FAQs provide a concise overview of the key concepts and methods related to calculating the bond order of SO42-. In the next section, we will explore advanced applications of bond order calculations in understanding the properties and reactivity of sulfate ion.
Tips for Calculating Bond Order of SO42-
This section provides practical tips to assist you in accurately calculating the bond order of sulfate ion (SO42-).
Tip 1: Understand Resonance Structures
Draw the resonance structures of SO42- to identify the electron delocalization and equivalent S-O bonds.
Tip 2: Assign Formal Charges
Assign formal charges to each atom in the resonance structures to determine the most stable structure and electron distribution.
Tip 3: Construct Molecular Orbitals
Construct molecular orbitals to visualize the electron distribution and bonding interactions within SO42-.
Tip 4: Consider Hybridization
Analyze the hybridization of the sulfur atom’s orbitals to understand the formation of sigma bonds and the tetrahedral molecular geometry.
Tip 5: Utilize Spectroscopic Techniques
Employ spectroscopic techniques, such as IR and Raman spectroscopy, to probe the vibrational modes and determine the bond order experimentally.
By following these tips, you can enhance your understanding of the electronic structure, bonding, and properties of sulfate ion. These insights are essential for predicting and controlling the reactivity of SO42- in various chemical and biological systems.
In the concluding section, we will explore advanced applications of bond order calculations in understanding the complex behavior of sulfate ion in diverse scientific fields.
Conclusion
Our detailed exploration of “how to calculate bond order of SO42-” has illuminated the fundamental concepts and advanced techniques involved in understanding the electronic structure and bonding of sulfate ion. By considering resonance structures, formal charges, molecular orbitals, and hybridization, we gained insights into the delocalization of electrons and the formation of equivalent S-O bonds.
The calculation of bond order is not merely an academic exercise; it has profound implications in various scientific fields. Understanding bond order enables us to predict and control the reactivity of sulfate ion in diverse chemical and biological systems. Moreover, it provides a foundation for exploring the complex behavior of sulfate ion in environmental, industrial, and medical applications.
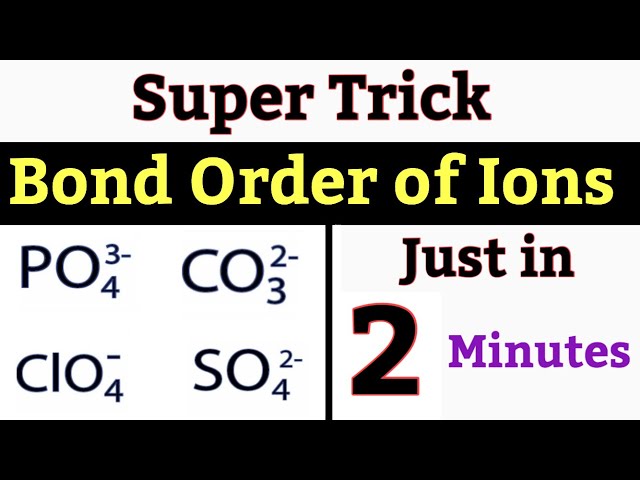