Bond order resonance is a computational method used to estimate the bond order between atoms in a molecule.
Understanding bond order resonance is essential for predicting the stability, reactivity, and properties of chemical compounds. Resonance structures are often used to represent the electronic structure of molecules, and bond order resonance provides a quantitative measure of the relative importance of these structures.
This article will explore the steps involved in calculating bond order resonance, including the use of resonance theory and quantum mechanics.
How to Calculate Bond Order Resonance
Understanding the aspects of bond order resonance is fundamental to comprehend its calculation. Key aspects include:
- Resonance structures
- Resonance hybrid
- Bond order
- Electronegativity
- Molecular orbitals
- Quantum mechanics
- Chemical bonding
- Molecular stability
- Chemical reactivity
- Molecular properties
These aspects are interconnected. Resonance structures provide a qualitative description of the electronic structure of a molecule, while bond order resonance provides a quantitative measure of the relative importance of these structures. Quantum mechanics provides the theoretical framework for understanding resonance and bond order. Electronegativity, molecular orbitals, and chemical bonding are all factors that influence bond order resonance. Ultimately, understanding bond order resonance is essential for predicting the stability, reactivity, and properties of chemical compounds.
Resonance structures
Resonance structures are a fundamental concept in chemistry, used to describe the electronic structure of molecules. They are particularly important in understanding how to calculate bond order resonance, which is a measure of the strength of the bond between two atoms.
Resonance structures arise when there are multiple possible Lewis structures for a molecule. These structures differ in the placement of the electrons, but they all have the same number of valence electrons and the same overall charge. For example, the carbonate ion (CO32-) has three resonance structures, as shown below:
O=C-O- O-C=O- O-C-O- | | | O O O
The bond order resonance between the carbon and oxygen atoms in the carbonate ion is calculated by taking the average of the bond orders in the three resonance structures. In this case, the bond order resonance is 1.33, which indicates that the bond between the carbon and oxygen atoms is stronger than a single bond but weaker than a double bond.
Understanding bond order resonance is essential for predicting the stability, reactivity, and properties of chemical compounds. For example, compounds with higher bond order resonances are generally more stable and less reactive. Bond order resonance can also be used to explain the geometry of molecules and the strength of their bonds.
Resonance hybrid
In the context of calculating bond order resonance, resonance hybrids are crucial for understanding the electronic structure of molecules and their properties. A resonance hybrid is a hypothetical structure that represents the average of all possible resonance structures for a molecule. It combines the features of all the resonance structures, taking into account their relative contributions.
- Weighted average: A resonance hybrid is a weighted average of all resonance structures, where the weights are determined by the stability of each structure.
- Intermediate properties: The properties of a resonance hybrid are intermediate between those of the contributing resonance structures.
- Delocalized electrons: In a resonance hybrid, the electrons are delocalized over the entire molecule, rather than being localized to specific atoms or bonds.
- Stability: Resonance hybrids are generally more stable than any of the individual resonance structures.
By considering resonance hybrids, chemists can gain a more accurate understanding of the electronic structure and properties of molecules. Resonance hybrids provide a valuable tool for predicting molecular properties, such as bond lengths, bond angles, and molecular polarity. They also help to explain the reactivity and stability of molecules.
Bond order
Bond order is a central concept in chemistry that describes the strength and type of chemical bond between two atoms. In the context of calculating bond order resonance, it plays a crucial role in determining the relative importance of different resonance structures and understanding the electronic structure of molecules.
- Bond length: Bond order is inversely related to bond length. Shorter bonds correspond to higher bond orders, indicating a stronger bond.
- Bond strength: Bond order is directly proportional to bond strength. Bonds with higher bond orders are stronger and less likely to break.
- Bond type: Bond order can be used to distinguish between different types of bonds, such as single bonds, double bonds, and triple bonds. Single bonds have a bond order of 1, double bonds have a bond order of 2, and triple bonds have a bond order of 3.
- Electron density: Bond order is related to the electron density between the bonded atoms. Higher bond orders correspond to higher electron density, indicating a greater overlap between the atomic orbitals.
Understanding bond order is essential for predicting the stability, reactivity, and properties of chemical compounds. By considering bond order resonance, chemists can gain a more accurate picture of the electronic structure of molecules and their behavior.
Electronegativity
Electronegativity is a fundamental property of atoms that influences their ability to attract electrons, thereby shaping the electronic structure and properties of molecules. In the context of calculating bond order resonance, electronegativity plays a crucial role in determining the distribution of electrons and the strength of chemical bonds.
- Electronegative Potential: Electronegative potential quantifies the tendency of an atom to attract electrons towards itself, determining the polarity of bonds and the overall charge distribution within a molecule.
- Pauling Scale: The Pauling scale is a widely used measure of electronegativity, assigning numerical values to different elements based on their ability to attract electrons. This scale provides a relative measure of electronegativity, aiding in the prediction of bond polarity and molecular properties.
- Electronegativity and Bond Length: Electronegativity influences bond length, as more electronegative atoms tend to form shorter and stronger bonds. This is due to the increased attraction between the electronegative atom and the shared electrons.
- Electronegativity and Resonance: Electronegativity affects resonance by stabilizing certain resonance structures over others, as more electronegative atoms tend to concentrate electron density towards themselves. This can influence the overall electronic structure and properties of the molecule.
Understanding electronegativity is crucial for calculating bond order resonance accurately, as it provides insights into the distribution of electrons and the strength of chemical bonds. By considering the electronegativities of the atoms involved, chemists can gain a more precise understanding of the electronic structure and properties of molecules.
Molecular orbitals
In the realm of calculating bond order resonance, molecular orbitals (MOs) emerge as a fundamental concept, providing a detailed account of the electronic structure of molecules. These orbitals are mathematical functions that describe the wave-like behavior of electrons within a molecule, encompassing their energy, spatial distribution, and interactions.
- Function: MOs are represented by mathematical functions () that describe the wave-like behavior of electrons, capturing their energy and spatial distribution.
- Electron Density: MOs provide insights into electron density distribution within a molecule, indicating the probability of finding electrons in specific regions.
- Bonding and Antibonding: MOs can be classified as bonding or antibonding, depending on their effect on the overlap and strength of chemical bonds.
- Molecular Symmetry: The symmetry of MOs aligns with the symmetry of the molecule, allowing for the prediction of molecular properties and spectroscopic behavior.
Comprehending molecular orbitals is crucial for accurately calculating bond order resonance. By considering the energy levels, spatial distributions, and interactions of electrons within MOs, chemists gain a deeper understanding of the electronic structure of molecules, enabling precise predictions of bond order resonance and various molecular properties.
Quantum mechanics
Quantum mechanics plays a crucial role in understanding and calculating bond order resonance. It provides the theoretical framework for describing the electronic structure of molecules and the interactions between electrons. By applying the principles of quantum mechanics, chemists can accurately predict the bond order resonance of a molecule, which is essential for understanding its stability, reactivity, and properties.
One of the key concepts in quantum mechanics is the wave-particle duality of electrons. Electrons can behave both as particles and as waves, and their behavior is described by wave functions. The wave function of an electron provides information about its energy, momentum, and spatial distribution. In the context of bond order resonance, the wave function of an electron can be used to determine the probability of finding the electron in a particular region of space. This information is essential for calculating the bond order resonance, as it allows chemists to determine the extent to which the electrons are delocalized over the molecule.
Another important concept in quantum mechanics is the Pauli exclusion principle. This principle states that no two electrons can occupy the same quantum state. In the context of bond order resonance, the Pauli exclusion principle can be used to determine the number of electrons that can occupy a particular molecular orbital. This information is essential for calculating the bond order resonance, as it allows chemists to determine the number of bonds that can be formed between two atoms.
Quantum mechanics is a powerful tool for understanding and calculating bond order resonance. By applying the principles of quantum mechanics, chemists can gain a deeper understanding of the electronic structure of molecules and their properties.
Chemical bonding
Chemical bonding is the interaction between atoms or molecules that enables the formation of chemical substances that contain two or more atoms. The strength of chemical bonding influences various properties of a molecule, including its stability, reactivity, and physical properties. Understanding chemical bonding is essential for accurately calculating bond order resonance, as it provides insights into the nature and strength of the bonds between atoms.
- Types of Bonds: Chemical bonds can be classified into various types, including covalent bonds, ionic bonds, and metallic bonds. Each type of bond has its own unique characteristics and properties, which affect the bond order resonance.
- Bond Strength: The strength of a chemical bond is determined by the amount of energy required to break the bond. Bond strength is directly related to bond order resonance, as stronger bonds typically have higher bond order resonance values.
- Bond Length: Bond length is the distance between the nuclei of two bonded atoms. Bond length is inversely related to bond order resonance, as shorter bonds generally have higher bond order resonance values.
- Molecular Geometry: The arrangement of atoms in a molecule is determined by the type and strength of the chemical bonds between them. Bond order resonance can influence the molecular geometry by affecting the bond lengths and bond angles.
In summary, chemical bonding plays a crucial role in determining the bond order resonance of a molecule. By understanding the different types of chemical bonds, their strengths, and their effects on bond length and molecular geometry, chemists can accurately calculate bond order resonance values, which provide valuable insights into the electronic structure and properties of molecules.
Molecular stability
In the context of calculating bond order resonance, molecular stability plays a critical role in understanding the electronic structure and properties of molecules. Bond order resonance provides insights into the relative stability of different resonance structures, which contributes to the overall stability of the molecule.
- Bond strength: The strength of the bonds between atoms affects the stability of a molecule. Bond order resonance can help determine the strength of bonds, as stronger bonds typically have higher bond order resonance values.
- Resonance energy: The resonance energy of a molecule is the energy difference between the actual molecule and the hypothetical molecule with the lowest energy resonance structure. Higher resonance energy indicates greater stability, as the molecule is more likely to adopt resonance structures that lower its energy.
- Molecular geometry: The arrangement of atoms in a molecule can influence its stability. Bond order resonance can provide insights into the preferred molecular geometry by indicating the most stable resonance structures.
- Reactivity: The reactivity of a molecule is related to its stability. More stable molecules are generally less reactive, as they are less likely to undergo chemical reactions that would disrupt their stable electronic structure.
Understanding molecular stability is essential for accurately calculating bond order resonance. By considering the various factors that contribute to molecular stability, chemists can gain a deeper understanding of the electronic structure and properties of molecules, and make more accurate predictions about their behavior.
Chemical reactivity
Chemical reactivity is a crucial aspect of “how to calculate bond order resonance” as it provides insights into the behavior and stability of molecules. It refers to the tendency of a molecule to undergo chemical reactions, forming new substances with different properties.
- Reaction Rates: Bond order resonance can influence the rate at which a molecule reacts with other species. Molecules with higher bond order resonance values tend to react more slowly, as the delocalized electrons are less available for bond formation with other molecules.
- Activation Energy: The activation energy of a reaction is the minimum amount of energy required for the reaction to occur. Bond order resonance can affect the activation energy by stabilizing the transition state of the reaction. A more stable transition state leads to a lower activation energy and, consequently, a faster reaction rate.
- Selectivity: Bond order resonance can influence the selectivity of a reaction, which refers to the preference of a molecule to react with one type of molecule over another. By considering the resonance structures of the reactants and products, chemists can predict the most favorable reaction pathway.
- Regioselectivity: Regioselectivity refers to the preference of a molecule to react at a specific site. Bond order resonance can provide insights into the regioselectivity of a reaction by indicating the most reactive site on a molecule.
In summary, chemical reactivity is an important factor to consider when calculating bond order resonance, as it provides valuable information about the stability, reaction rates, selectivity, and regioselectivity of molecules. Understanding chemical reactivity enables chemists to make more accurate predictions about the behavior of molecules and design molecules with desired properties.
Molecular properties
Molecular properties play a crucial role in understanding the chemical behavior and applications of molecules. By calculating bond order resonance, chemists can gain insights into various molecular properties that determine how molecules interact with each other and their surroundings.
- Dipole moment: Dipole moment measures the polarity of a molecule, indicating the uneven distribution of electrical charge. Bond order resonance can influence the dipole moment by affecting the distribution of electrons within the molecule.
- Polarizability: Polarizability describes how easily a molecule’s electron cloud can be distorted by an external electric field. Bond order resonance can affect polarizability by influencing the delocalization of electrons.
- Magnetic susceptibility: Magnetic susceptibility measures the response of a molecule to a magnetic field. Bond order resonance can affect magnetic susceptibility by influencing the number and type of unpaired electrons in the molecule.
- Optical properties: Optical properties, such as color and absorption spectra, are influenced by the electronic structure of a molecule. Bond order resonance can affect optical properties by altering the energy levels and transitions of electrons.
In summary, understanding molecular properties is essential for accurately calculating bond order resonance. By considering the various molecular properties outlined above, chemists can gain a deeper understanding of the electronic structure and behavior of molecules.
Frequently Asked Questions about Bond Order Resonance
This FAQ section addresses common questions and clarifies key aspects of “how to calculate bond order resonance”.
Question 1: What is bond order resonance?
Bond order resonance is a method for calculating the bond order between atoms in a molecule, taking into account the resonance structures of the molecule.
Question 2: Why is bond order resonance important?
Bond order resonance provides valuable insights into the electronic structure and properties of molecules, such as their stability, reactivity, and molecular properties.
Question 3: What are resonance structures?
Resonance structures are different Lewis structures for the same molecule that have the same number of valence electrons and the same overall charge. They represent the delocalization of electrons within the molecule.
Question 4: How do you calculate bond order resonance?
Bond order resonance is calculated by taking the average of the bond orders in the resonance structures of the molecule.
Question 5: What factors affect bond order resonance?
Bond order resonance is influenced by factors such as electronegativity, molecular orbitals, and quantum mechanics.
Question 6: How does bond order resonance relate to molecular properties?
Bond order resonance provides insights into various molecular properties, including dipole moment, polarizability, magnetic susceptibility, and optical properties.
In summary, understanding bond order resonance is crucial for gaining insights into the electronic structure and behavior of molecules. By considering the factors that affect bond order resonance, chemists can make accurate predictions about molecular properties and chemical reactivity.
In the next section, we will explore advanced applications of bond order resonance in chemistry.
Tips for Calculating Bond Order Resonance
Accurately calculating bond order resonance is crucial for understanding the electronic structure and properties of molecules. Here are some valuable tips to help you master this technique:
Tip 1: Understand Resonance Structures
Identify and analyze the resonance structures of the molecule to gain insights into the delocalization of electrons.
Tip 2: Calculate Bond Orders
Determine the bond orders in each resonance structure using appropriate methods, such as the Pauling formula or molecular orbital theory.
Tip 3: Consider Electronegativity
Take into account the electronegativity of the atoms involved, as it influences the distribution of electrons and bond strength.
Tip 4: Utilize Quantum Mechanics
Apply the principles of quantum mechanics, such as wave-particle duality and the Pauli exclusion principle, to understand electron behavior and bond formation.
Tip 5: Analyze Molecular Geometry
Consider the molecular geometry and symmetry to determine the most stable resonance structures and their impact on bond order resonance.
Summary: By following these tips, you can effectively calculate bond order resonance and gain valuable insights into the electronic structure, stability, and properties of molecules.
In the concluding section of this article, we will explore advanced applications of bond order resonance in chemistry, showcasing its significance in understanding complex molecular systems.
Conclusion
In this article, we have explored the intricacies of “how to calculate bond order resonance”. We have gained insights into the fundamental concepts, such as resonance structures, bond orders, and the influence of factors like electronegativity and molecular geometry. By understanding bond order resonance, chemists can accurately predict the stability, reactivity, and properties of molecules.
Key points to remember:
- Bond order resonance provides a quantitative measure of the relative importance of resonance structures.
- It is crucial for understanding the electronic structure, stability, and properties of molecules.
- Factors such as electronegativity, molecular orbitals, and quantum mechanics play significant roles in determining bond order resonance.
Understanding bond order resonance is not merely an academic exercise but a powerful tool for unraveling the mysteries of molecular behavior. It empowers chemists to design new materials, optimize chemical reactions, and develop innovative technologies that shape our world. As we continue to push the boundaries of chemistry, the significance of bond order resonance will only grow, enabling us to harness the power of molecules for the betterment of society.
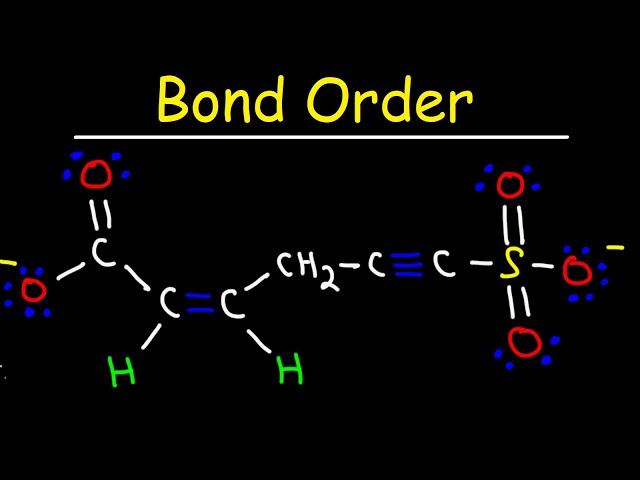