How to Calculate the Bond Order of N2: A Comprehensive Guide
In chemistry, bond order is a crucial concept for understanding the properties and reactivity of molecules. The bond order of dinitrogen (N2) determines its stability, strength, and involvement in various chemical reactions, making its calculation a key task in understanding nitrogen chemistry.
In this article, we will explore the significance of bond order in N2, its relevance to chemical bonding, and a historical perspective on its development. We will then provide a step-by-step guide on calculating the bond order of N2 using established theoretical approaches, ensuring a comprehensive understanding for readers of all levels.
How to Calculate the Bond Order of N2
Calculating the bond order of dinitrogen (N2) is essential for comprehending its chemical bonding and reactivity. Key aspects to consider include:
- Molecular orbital theory
- Electron configuration
- Bond length
- Bond energy
- Magnetic properties
- Thermodynamic stability
- Chemical reactivity
- Industrial applications
- Biological significance
- Historical development
Understanding these aspects provides insights into the nature of the N2 bond, its role in various chemical processes, and its importance in fields such as fertilizer production, atmospheric chemistry, and biological nitrogen fixation.
Molecular Orbital Theory
Molecular orbital theory is a fundamental concept in chemistry that describes the electronic structure of molecules. It provides a framework for understanding the bonding, properties, and reactivity of molecules by considering the wave-like behavior of electrons within the molecule. In the context of calculating the bond order of N2, molecular orbital theory plays a crucial role.
The bond order of a molecule is a measure of the strength of the bond between two atoms. It is calculated based on the number of electrons occupying the bonding and antibonding molecular orbitals. In the case of N2, the bond order can be determined using molecular orbital theory by considering the electronic configuration of the molecule and the symmetry of the molecular orbitals.
The molecular orbital diagram of N2 shows that the bonding molecular orbital is filled with four electrons, while the antibonding molecular orbital is empty. This results in a bond order of three, indicating a strong triple bond between the two nitrogen atoms. The stability and unreactivity of N2 can be attributed to this strong bond order.
Electron Configuration
Electron configuration plays a crucial role in calculating the bond order of N2. The bond order, which reflects the strength of the bond between the two nitrogen atoms, is directly affected by the number and arrangement of electrons in the molecular orbitals of N2. The electron configuration of N2 is 1s22s22p6, which means that each nitrogen atom contributes five valence electrons to the bond. These valence electrons occupy the molecular orbitals formed by the overlap of the atomic orbitals of the two nitrogen atoms.
The molecular orbital diagram of N2 shows that the bonding molecular orbital, denoted as 2p, is filled with four electrons, while the antibonding molecular orbital, denoted as 2p*, is empty. This results in a bond order of three, indicating a strong triple bond between the two nitrogen atoms. The stability and unreactivity of N2 can be attributed to this strong bond order, which is a consequence of its electron configuration.
Understanding the relationship between electron configuration and bond order is essential for predicting and explaining the properties and reactivity of molecules. In the case of N2, the strong triple bond resulting from its electron configuration makes it a relatively inert molecule, explaining its low reactivity and abundance in the Earth’s atmosphere.
Bond length
Bond length, defined as the equilibrium distance between the nuclei of two bonded atoms, plays a critical role in calculating the bond order of N2. The bond order, which reflects the strength and stability of the bond, is inversely related to the bond length. A shorter bond length typically indicates a stronger bond and a higher bond order.
In the case of N2, the bond length is 109.76 pm, which corresponds to a bond order of three. This strong bond order is a consequence of the short bond length, which results from the effective overlap of the nitrogen atomic orbitals and the formation of a strong triple bond. The short bond length in N2 contributes to its stability and low reactivity, making it an inert gas under ambient conditions.
Understanding the relationship between bond length and bond order is crucial for predicting and explaining the properties and reactivity of molecules. In the context of N2, the short bond length and high bond order provide insights into its unique stability and its role in various chemical processes, such as fertilizer production and atmospheric chemistry.
Bond energy
Bond energy is a critical component of calculating the bond order of N2. Bond order, which reflects the strength and stability of the bond, is directly proportional to the bond energy. The bond energy of N2 is 945.4 kJ/mol, indicating a very strong triple bond between the two nitrogen atoms. This high bond energy is a consequence of the strong overlap of the nitrogen atomic orbitals and the formation of a stable electron configuration.
The bond energy of N2 plays a crucial role in its stability and low reactivity. The high bond energy makes N2 an inert gas under ambient conditions, and it requires a significant amount of energy to break the triple bond. This explains why N2 is abundant in the Earth’s atmosphere and why it is not readily involved in chemical reactions.
Understanding the relationship between bond energy and bond order is essential for predicting and explaining the properties and reactivity of molecules. In the case of N2, the high bond energy and bond order provide insights into its unique stability and its role in various chemical processes, such as fertilizer production and atmospheric chemistry.
Magnetic properties
Magnetic properties play a crucial role in understanding the bond order of N2. The magnetic properties of a molecule are determined by the number of unpaired electrons it possesses. N2 has no unpaired electrons, making it diamagnetic. This diamagnetic nature is a consequence of the triple bond between the two nitrogen atoms, which results in all electrons being paired.
- Susceptibility
The magnetic susceptibility of N2 is negative, indicating its diamagnetic nature. This negative susceptibility means that N2 is repelled by magnetic fields.
- Magnetic moment
The magnetic moment of N2 is zero due to the absence of unpaired electrons. This zero magnetic moment further confirms the diamagnetic nature of N2.
- NMR spectroscopy
N2 is NMR inactive due to its lack of nuclear spin. This NMR inactivity is a consequence of the diamagnetic nature of N2 and the absence of unpaired electrons.
- EPR spectroscopy
N2 is EPR inactive due to the absence of unpaired electrons. This EPR inactivity further supports the diamagnetic nature of N2 and provides additional evidence for the triple bond between the two nitrogen atoms.
The magnetic properties of N2, such as its diamagnetic nature, zero magnetic moment, NMR inactivity, and EPR inactivity, are all consistent with the triple bond between the two nitrogen atoms. These magnetic properties provide valuable insights into the electronic structure and bonding of N2, complementing other methods for calculating the bond order.
Thermodynamic stability
Thermodynamic stability is a crucial aspect of calculating the bond order of N2. The bond order, which reflects the strength and stability of the bond between the two nitrogen atoms, is directly related to the thermodynamic stability of the molecule. A higher bond order typically corresponds to a more stable molecule.
In the case of N2, the triple bond between the two nitrogen atoms results in a very stable molecule. This stability is reflected in the high bond energy (945.4 kJ/mol) and the low bond length (109.76 pm) of N2. The strong bond between the nitrogen atoms makes N2 unreactive under ambient conditions, and it requires a significant amount of energy to break the bond.
Understanding the relationship between bond order and thermodynamic stability is essential for predicting and explaining the properties and reactivity of molecules. In the context of N2, the high bond order and thermodynamic stability provide insights into its unique properties, such as its inertness and abundance in the Earth’s atmosphere.
The calculation of bond order, considering thermodynamic stability, has practical applications in various fields, including chemistry, materials science, and atmospheric chemistry. By understanding the bond order and thermodynamic stability of molecules, scientists can design and develop new materials with desired properties, predict the reactivity of molecules in chemical reactions, and monitor atmospheric processes.
Chemical reactivity
Chemical reactivity is a crucial aspect to consider when calculating the bond order of dinitrogen (N2). It provides insights into the behavior and properties of N2, influencing its stability, reactivity, and applications. Here are several key components to consider:
- Bond strength
The bond order of N2 is directly related to its bond strength. A higher bond order indicates a stronger bond, which in turn affects the chemical reactivity of N2. A strong triple bond, as in the case of N2, makes it less reactive compared to molecules with weaker bonds.
- Activation energy
The activation energy of a chemical reaction is the minimum amount of energy required to initiate the reaction. The bond order of N2 influences its activation energy. A higher bond order typically corresponds to a higher activation energy, making it more difficult to break the bond and initiate reactions.
- Reaction pathways
The bond order of N2 can influence the reaction pathways available to it. For example, the strong triple bond in N2 makes it less likely to undergo certain types of reactions, such as addition reactions, compared to molecules with weaker bonds.
- Catalytic processes
Catalysts are substances that can increase the rate of a chemical reaction without being consumed. The bond order of N2 can affect the effectiveness of catalysts. In some cases, a higher bond order can make it more challenging for catalysts to facilitate reactions involving N2.
Understanding the chemical reactivity of N2, in conjunction with its bond order, is essential for predicting its behavior in various chemical processes. This knowledge finds applications in diverse fields, including atmospheric chemistry, industrial processes, and fertilizer production, where controlling and manipulating the reactivity of N2 is crucial.
Industrial applications
The calculation of bond order plays a critical role in understanding the behavior and properties of molecules, which has significant implications for industrial applications. In the context of dinitrogen (N2), its bond order is a crucial factor in determining its reactivity and suitability for various industrial processes.
One prominent industrial application of N2 is in the production of fertilizers. Ammonia (NH3), a key component in fertilizer production, is synthesized through the Haber-Bosch process, which involves the reaction of N2 with hydrogen (H2). The strong triple bond in N2 makes it relatively unreactive, and calculating its bond order helps determine the optimal conditions for the Haber-Bosch process to achieve high ammonia yield.
Furthermore, the bond order of N2 is essential in understanding its behavior in combustion processes. In internal combustion engines, N2 acts as a diluent, reducing the temperature and controlling the rate of combustion. Calculating the bond order of N2 provides insights into its thermal stability and helps optimize engine performance and efficiency.
In summary, calculating the bond order of N2 is a critical aspect of industrial applications, from fertilizer production to combustion processes. Understanding the bond order enables the optimization of these processes, leading to improved efficiency, reduced costs, and enhanced product quality.
Biological significance
The calculation of the bond order of dinitrogen (N2) has profound implications for understanding its biological significance and its role in various biochemical processes. N2 is essential for life on Earth, as it serves as the primary source of nitrogen for biological systems.
Nitrogen is a crucial component of proteins, nucleic acids, and other biomolecules. The strong triple bond in N2 makes it relatively unreactive, and calculating its bond order helps elucidate the mechanisms by which nitrogen is fixed and incorporated into biological compounds. This understanding is fundamental for studying nitrogen metabolism, enzymatic reactions, and the nitrogen cycle.
One significant application of calculating the bond order of N2 in biological systems is in the study of nitrogen fixation. Nitrogen-fixing bacteria possess specialized enzymes called nitrogenases that can break the strong triple bond in N2 and convert it into ammonia (NH3). This process is essential for the synthesis of amino acids, proteins, and other nitrogen-containing biomolecules.
In summary, calculating the bond order of N2 provides valuable insights into its biological significance, enabling researchers to explore nitrogen metabolism, enzymatic reactions, and the nitrogen cycle. This understanding has practical applications in agriculture, biotechnology, and environmental science.
Historical development
The historical development of scientific understanding and the advancement of experimental techniques have played a crucial role in shaping how we calculate and comprehend the bond order of dinitrogen (N2). Early theoretical models and experimental observations laid the foundation for the current methods used to determine the bond order of N2.
One significant historical development was the development of molecular orbital theory in the early 20th century. This theory provided a framework for understanding the electronic structure of molecules and laid the groundwork for calculating bond orders. The application of molecular orbital theory to N2 helped establish the triple bond nature of the molecule and provided insights into its stability and reactivity.
Furthermore, experimental techniques such as X-ray crystallography and spectroscopy have been instrumental in validating theoretical models and providing experimental evidence for the bond order of N2. By measuring bond lengths, vibrational frequencies, and other physical properties, scientists have been able to corroborate the theoretical predictions and gain a deeper understanding of the bonding in N2.
Frequently Asked Questions
This section addresses frequently asked questions and clarifies common misconceptions regarding the calculation of bond order in N2:
Question 1: What is the significance of bond order in N2?
Answer: Bond order determines the strength and stability of the N2 molecule, influencing its reactivity, thermodynamic properties, and involvement in various chemical processes.
Question 2: How is bond order calculated for N2?
Answer: Bond order is calculated using molecular orbital theory, considering the number of electrons in bonding and antibonding molecular orbitals. In N2, the bond order is three, indicating a triple bond.
Question 3: What experimental techniques validate the calculated bond order of N2?
Answer: Techniques like X-ray crystallography and spectroscopy provide experimental evidence for bond lengths, vibrational frequencies, and other properties, corroborating theoretical bond order calculations.
Question 4: How does bond order affect the reactivity of N2?
Answer: The strong triple bond in N2 (bond order of 3) makes it relatively unreactive, requiring high activation energy to break the bond and initiate reactions.
Question 5: What is the industrial significance of N2 bond order?
Answer: Understanding bond order is crucial in optimizing industrial processes like fertilizer production (Haber-Bosch process) and combustion in internal combustion engines.
Question 6: Why is N2 bond order important in biological systems?
Answer: N2 bond order is essential for studying nitrogen metabolism, enzymatic reactions, and the nitrogen cycle, as it influences nitrogen fixation and the incorporation of nitrogen into biomolecules.
These FAQs provide key insights into the calculation, significance, and implications of bond order in N2, laying the groundwork for further exploration of its chemical and biological properties.
Transition to the next article section: In the following section, we will delve deeper into the applications of bond order calculations in various fields, exploring how this concept guides our understanding of molecular behavior and reactivity.
TIPS
This section provides practical tips and strategies to enhance the accuracy and efficiency of bond order calculations in N2, empowering researchers and students to refine their understanding of this crucial molecular property.
Tip 1: Utilize advanced computational methods: Employ sophisticated computational methods like density functional theory (DFT) and Hartree-Fock (HF) theory for precise bond order calculations, considering electron correlation and exchange effects.
Tip 2: Consider relativistic effects: For heavy atoms or molecules with strong relativistic effects, incorporate relativistic corrections into the calculations to account for the influence of relativity on bond order.
Tip 3: Optimize basis set selection: Choose an appropriate basis set that provides a balanced representation of the molecular orbitals involved in the N2 bond, ensuring accurate bond order determination.
Tip 4: Employ experimental data validation: Validate the calculated bond order using experimental data such as bond lengths and vibrational frequencies obtained from spectroscopy or X-ray crystallography.
Tip 5: Understand the limitations of the method: Recognize the limitations of the chosen method and consider alternative approaches or complementary techniques to strengthen the reliability of the bond order calculations.
By implementing these tips, researchers can refine their bond order calculations, leading to a deeper understanding of the electronic structure and properties of N2, with broader implications in chemistry, physics, and materials science.
In the concluding section, we will explore the broader applications of bond order calculations, showcasing how this concept serves as a cornerstone for unraveling the intricacies of molecular behavior and reactivity.
Conclusion
This article provided a comprehensive exploration of how to calculate the bond order of N2, a crucial concept in chemistry. Understanding bond order is essential for comprehending the stability, reactivity, and properties of N2, which plays a vital role in various chemical processes and biological systems.
Key insights gained from this exploration include:
- Bond order is a measure of the strength and stability of a chemical bond, calculated based on the number of electrons in bonding and antibonding molecular orbitals.
- In N2, the bond order is three, indicating a strong triple bond that contributes to its inertness and low reactivity.
- Bond order is influenced by a range of factors, including molecular orbital theory, electron configuration, bond length, bond energy, magnetic properties, thermodynamic stability, chemical reactivity, and biological significance.
Comprehending how to calculate bond order empowers scientists and researchers to delve deeper into the electronic structure and properties of molecules, paving the way for advancements in diverse fields such as chemistry, materials science, and biochemistry. By unlocking the secrets of bond order, we can continue to unravel the mysteries of the molecular world and harness its potential for innovation and progress.
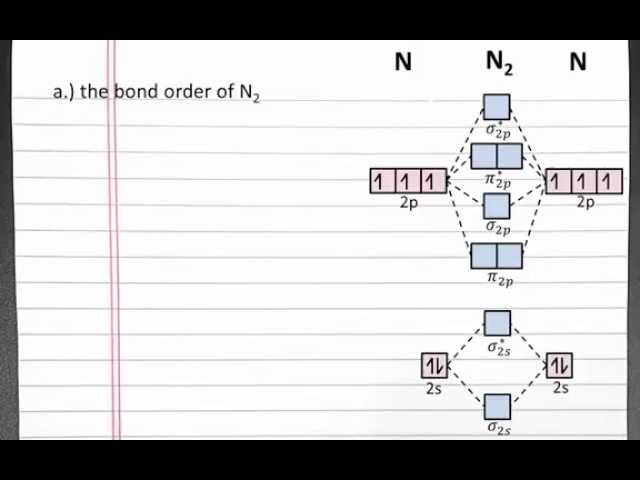