Bond order is a crucial concept in chemistry that measures the strength and stability of chemical bonds. Determining bond order from molecular electron configuration involves analyzing the distribution of electrons in a molecule’s molecular orbitals, providing valuable insights into its chemical properties.
Understanding bond order is essential for predicting molecular geometry, polarity, and reactivity. For instance, in the case of diatomic molecules, higher bond orders correspond to shorter bond lengths and stronger bonds. The concept originated from Linus Pauling’s pioneering work on valence bond theory, revolutionizing our comprehension of molecular bonding.
This article delves into the detailed steps to determine bond order from molecular electron configuration, exploring the underlying principles and applications in various chemical contexts.
How to Determine Bond Order from Molecular Electron Configuration
Determining bond order from molecular electron configuration is essential in understanding chemical bonding and molecular structure. Key aspects include:
- Molecular orbital theory
- Electron distribution
- Bonding and antibonding orbitals
- Valence electrons
- Paramagnetism
- Resonance structures
- Hybridization
- Delocalization
- Formal charge
- Bond length
Understanding these aspects allows chemists to predict molecular properties, reactivity, and behavior. By analyzing the molecular electron configuration, one can determine the number and type of bonds present, their strength, and the overall stability of the molecule.
Molecular orbital theory
Molecular orbital theory (MOT) is a fundamental concept that underpins our understanding of chemical bonding and the electronic structure of molecules. It provides a theoretical framework for determining bond order from molecular electron configuration, offering insights into the strength and stability of chemical bonds.
MOT describes the behavior of electrons in molecules by considering them to occupy specific molecular orbitals, which are mathematical functions that describe the wave-like properties of electrons. These orbitals are formed by the combination of atomic orbitals, and their energies and shapes determine the chemical properties of the molecule. By analyzing the molecular orbital configuration, one can determine the number and type of bonds present, as well as their strength and overall stability.
For instance, in the case of diatomic molecules, the bond order is directly related to the number of bonding electrons in the molecular orbital diagram. A higher bond order indicates a stronger bond, as it corresponds to a greater number of bonding electrons and a lower energy state. This understanding is crucial in predicting molecular properties such as bond length, bond strength, and reactivity.
Electron distribution
Electron distribution plays a critical role in determining bond order from molecular electron configuration. The bond order, which measures the strength and stability of a chemical bond, is directly influenced by the distribution of electrons within the molecule’s molecular orbitals.
In molecular orbital theory, electrons occupy specific molecular orbitals, which are formed by the combination of atomic orbitals. The number and type of molecular orbitals depend on the number of atoms and the type of bonds in the molecule. By analyzing the molecular orbital configuration, one can determine the electron distribution and hence the bond order.
For instance, in a diatomic molecule like hydrogen (H2), the two electrons occupy the bonding molecular orbital, resulting in a bond order of 1. This indicates a single bond between the two hydrogen atoms. In contrast, in a molecule like oxygen (O2), the electrons occupy both bonding and antibonding molecular orbitals, resulting in a bond order of 2. This indicates a double bond between the two oxygen atoms.
Understanding electron distribution and its impact on bond order has practical applications in various fields of science and engineering. For example, in materials science, it is used to design and develop new materials with specific properties. In biochemistry, it helps researchers understand the structure and function of biological molecules like proteins and DNA.
Bonding and antibonding orbitals
Bonding and antibonding orbitals are fundamental concepts in molecular orbital theory, which is used to describe the electronic structure of molecules and determine bond order. Bonding orbitals result from the constructive interference of atomic orbitals, leading to electron density concentrated between the atomic nuclei. This concentration strengthens the bond between the atoms. Conversely, antibonding orbitals arise from the destructive interference of atomic orbitals, resulting in electron density depleted between the atomic nuclei. This depletion weakens or even prevents bond formation.
In determining bond order from molecular electron configuration, bonding and antibonding orbitals play a crucial role. The bond order is directly related to the number of electrons occupying bonding and antibonding orbitals. A higher number of electrons in bonding orbitals and a lower number in antibonding orbitals result in a higher bond order, indicating a stronger bond. Conversely, a lower number of electrons in bonding orbitals and a higher number in antibonding orbitals result in a lower bond order, indicating a weaker bond or even no bond formation.
For example, in the case of the hydrogen molecule (H2), the two electrons occupy the bonding molecular orbital, resulting in a bond order of 1. This indicates a single bond between the two hydrogen atoms. In contrast, in the case of the oxygen molecule (O2), the electrons occupy both bonding and antibonding molecular orbitals, resulting in a bond order of 2. This indicates a double bond between the two oxygen atoms.
Understanding bonding and antibonding orbitals and their relationship with bond order is essential for chemists to predict molecular properties, reactivity, and behavior. It has practical applications in various fields, including materials science, biochemistry, and pharmaceutical chemistry.
Valence electrons
Valence electrons play a critical role in determining bond order from molecular electron configuration. Valence electrons are the electrons in the outermost shell of an atom, and they are responsible for chemical bonding. The number and type of valence electrons determine the chemical properties of an element and the types of bonds it can form.
In the context of determining bond order from molecular electron configuration, valence electrons are crucial because they participate in the formation of molecular orbitals. Molecular orbitals are formed by the overlap of atomic orbitals, and the number and type of molecular orbitals determine the bond order. For example, in a diatomic molecule like hydrogen (H2), the two valence electrons occupy the bonding molecular orbital, resulting in a bond order of 1. This indicates a single bond between the two hydrogen atoms.
Understanding the relationship between valence electrons and bond order has practical applications in various fields of chemistry. For instance, in materials science, it is used to design and develop new materials with specific properties. In biochemistry, it helps researchers understand the structure and function of biological molecules like proteins and DNA.
Paramagnetism
Paramagnetism is a form of magnetism that occurs when a material is attracted to a magnetic field. It is caused by the presence of unpaired electrons in the material’s atoms or molecules. Paramagnetism is an important consideration in determining bond order from molecular electron configuration because it can provide information about the number of unpaired electrons in a molecule.
In the context of determining bond order from molecular electron configuration, paramagnetism can be used to identify molecules with unpaired electrons. This is important because unpaired electrons can contribute to the bond order of a molecule. For example, in the case of the oxygen molecule (O2), the two unpaired electrons in the * antibonding molecular orbital contribute to the bond order of 2. This indicates a double bond between the two oxygen atoms.
Understanding the relationship between paramagnetism and bond order has practical applications in various fields of chemistry. For instance, in materials science, it is used to design and develop new materials with specific magnetic properties. In biochemistry, it helps researchers understand the structure and function of biological molecules like proteins and DNA.
In summary, paramagnetism is an important consideration in determining bond order from molecular electron configuration because it can provide information about the number of unpaired electrons in a molecule. This understanding has practical applications in various fields of chemistry, including materials science and biochemistry.
Resonance structures
Resonance structures are a crucial concept in determining bond order from molecular electron configuration. They provide a way to represent the delocalization of electrons in a molecule, which can affect the bond order. Delocalization occurs when electrons are not confined to a specific bond but are instead spread out over multiple atoms. This can lead to fractional bond orders, which are not possible to determine from the molecular electron configuration alone.
For example, the carbonate ion (CO3^2-) has three resonance structures. In each resonance structure, the double bond is located between different pairs of carbon and oxygen atoms. This delocalization of the double bond means that each carbon-oxygen bond has a bond order of 1.33, rather than 2. This fractional bond order is a result of the resonance between the three resonance structures.
Understanding resonance structures is essential for accurately determining bond order from molecular electron configuration. Resonance structures can also provide insights into the chemical properties of molecules. For example, molecules with delocalized electrons are often more stable and less reactive than molecules with localized electrons. This understanding has practical applications in various fields of chemistry, such as organic chemistry and materials science.
Hybridization
Hybridization is a fundamental concept in chemistry that describes the mixing of atomic orbitals to form new hybrid orbitals with different shapes and energies. It plays a crucial role in determining bond order from molecular electron configuration because the type of hybridization affects the geometry of the molecule and the number of bonds that can be formed.
For example, in the case of carbon, the 2s and three 2p orbitals hybridize to form four equivalent sp3 hybrid orbitals. These hybrid orbitals have a tetrahedral shape and can form four sigma bonds with other atoms. This hybridization is observed in molecules like methane (CH4), where the carbon atom is bonded to four hydrogen atoms with a bond order of 1 for each C-H bond.
Understanding hybridization is essential for accurately determining bond order from molecular electron configuration. It provides insights into the molecular geometry, bonding patterns, and overall stability of molecules. This understanding has practical applications in various fields, including organic chemistry, inorganic chemistry, and materials science.
Delocalization
Delocalization plays a significant role in determining bond order from molecular electron configuration. It refers to the dispersal of electrons over multiple atoms or molecular orbitals, rather than being confined to a specific bond. This delocalization can affect the bond order and overall stability of a molecule.
- Resonance
Resonance occurs when multiple Lewis structures can be drawn for a molecule, indicating the delocalization of electrons. The bond order in resonance structures is an average of the bond orders in each contributing structure.
- Aromatic Rings
Aromatic rings exhibit resonance due to the delocalization of pi electrons over the ring. This delocalization results in bond orders of 1.5 for each carbon-carbon bond in the ring.
- Hyperconjugation
Hyperconjugation is a type of delocalization involving the overlap of a sigma bond with an adjacent pi bond. It can increase the bond order of the sigma bond.
- Metal-Ligand Bonding
In coordination complexes, delocalization can occur between the metal d orbitals and the ligand orbitals. This delocalization affects the bond order and stability of the complex.
Delocalization can significantly alter the properties of molecules. Delocalized electrons are less reactive, leading to increased stability. Understanding delocalization is crucial for accurately determining bond order and predicting the chemical behavior of molecules.
Formal charge
Formal charge is a valuable tool in determining bond order from molecular electron configuration. It assigns a hypothetical charge to each atom in a molecule, providing insights into the distribution of electrons and the polarity of bonds.
- Definition
Formal charge is the hypothetical charge assigned to an atom in a molecule, calculated as the difference between the number of valence electrons in the free atom and the number of electrons assigned to that atom in the Lewis structure.
- Significance
Formal charge helps identify polar bonds and resonance structures. It enables the prediction of molecular properties such as stability, reactivity, and dipole moments.
- Applications
Formal charge is used in various chemical applications, including predicting the products of chemical reactions, determining the stability of intermediates, and understanding the electronic structure of complex molecules.
- Limitations
While formal charge provides valuable insights, it is important to recognize its limitations. It assumes a localized electron distribution and may not accurately represent the true charge distribution in all molecules.
In summary, formal charge is a useful concept that complements the determination of bond order from molecular electron configuration. It provides additional information about the distribution of electrons and the polarity of bonds, enhancing our understanding of molecular structure and properties.
Bond length
Determining bond order from molecular electron configuration involves understanding various factors, including bond length. Bond length, the distance between the nuclei of two bonded atoms, offers valuable insights into the strength and nature of chemical bonds.
- Atomic Radii
Atomic radii influence bond length. Larger atoms generally form longer bonds due to increased internuclear distance.
- Bond Order
Bond order and bond length are inversely related. Higher bond orders correspond to shorter bond lengths due to stronger bonding interactions.
- Hybridization
The hybridization of atomic orbitals affects bond length. For instance, sp3 hybridized orbitals lead to longer bonds compared to sp2 or sp hybridized orbitals.
- Electronegativity
Electronegativity differences between bonded atoms influence bond length. Bonds between atoms with significant electronegativity differences tend to be shorter.
Understanding bond length is crucial in determining bond order from molecular electron configuration. It provides insights into the molecular structure, stability, and reactivity. By analyzing bond lengths in combination with other factors, chemists can gain a deeper comprehension of chemical bonding and molecular behavior.
Frequently Asked Questions
This section addresses common questions and clarifies concepts related to determining bond order from molecular electron configuration.
Question 1: What is bond order and why is it important?
Answer: Bond order is a measure of the strength of a chemical bond. It indicates the number of electron pairs shared between two atoms and influences molecular properties such as bond length, stability, and reactivity.
Question 2: How do I use molecular electron configuration to determine bond order?
Answer: Examine the molecular orbital diagram and count the number of bonding and antibonding electrons. The bond order is half the difference between these counts.
Question 3: What is the relationship between bond order and bond length?
Answer: Bond order and bond length are inversely related. Higher bond orders correspond to shorter bond lengths due to stronger bonding interactions.
Question 4: How does hybridization affect bond order?
Answer: Hybridization influences the overlap and bonding interactions between atomic orbitals. Different hybridization types can result in varying bond orders.
Question 5: What are some examples of molecules with different bond orders?
Answer: Ethene (C2H4) has a C=C double bond (bond order 2), while benzene (C6H6) exhibits resonance with alternating single and double bonds (bond order 1.5).
Question 6: How can I apply this knowledge to predict molecular properties?
Answer: Understanding bond order enables the prediction of molecular geometry, bond strength, and reactivity, providing valuable insights into chemical behavior.
These FAQs provide a concise overview of key concepts related to determining bond order from molecular electron configuration. In the following section, we will delve deeper into advanced applications and explore the significance of bond order in various chemical contexts.
Tips for Determining Bond Order from Molecular Electron Configuration
To enhance your understanding and proficiency in determining bond order from molecular electron configuration, consider these practical tips:
Tip 1: Systematically analyze the molecular orbital diagram, identifying the number of bonding and antibonding electrons.
Tip 2: Utilize the formula: Bond Order = (Number of Bonding Electrons – Number of Antibonding Electrons) / 2.
Tip 3: Pay attention to exceptions, such as paramagnetic molecules with unpaired electrons, which may affect the bond order.
Tip 4: Consider resonance structures, which can involve delocalization of electrons and fractional bond orders.
Tip 5: Understand the influence of hybridization on bond order, as it affects the overlap and interactions of atomic orbitals.
Tip 6: Utilize formal charges to assess the distribution of electrons and polarity of bonds, which can provide insights into bond order.
Tip 7: Relate bond order to bond length, as higher bond orders typically correspond to shorter bond lengths due to stronger bonding interactions.
Tip 8: Apply these principles to predict molecular properties, such as stability, reactivity, and bond strength, based on the determined bond order.
By incorporating these tips into your approach, you will enhance your accuracy and deepen your understanding of chemical bonding.
In the next section, we will explore advanced applications of bond order, demonstrating its significance in various chemical contexts.
Conclusion
In summary, determining bond order from molecular electron configuration provides valuable insights into the nature of chemical bonds. By analyzing molecular orbital diagrams and considering factors such as resonance, hybridization, and electronegativity, we can accurately determine bond order and gain a deeper understanding of molecular structure and properties.
This understanding is crucial for predicting molecular behavior, reactivity, and stability. It enables chemists to design and synthesize new materials with tailored properties, develop pharmaceuticals with specific therapeutic effects, and understand the intricate interactions within biological systems. The determination of bond order from molecular electron configuration remains a fundamental tool in advancing our knowledge of chemistry and its applications.
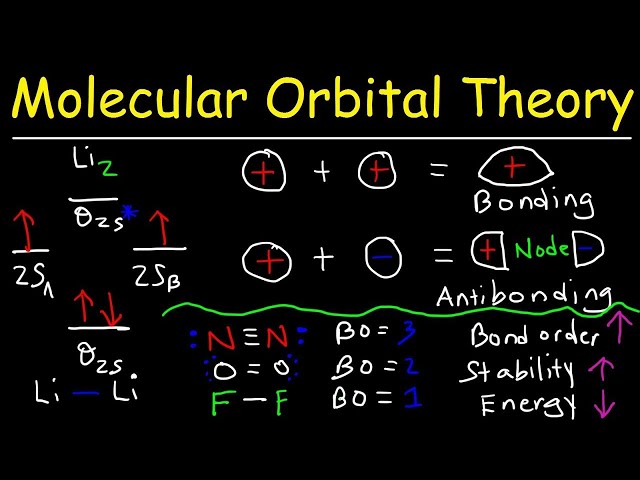