Understanding “How to Determine Bond Order from Structure” is a crucial skill for comprehending molecular bonding.
Determining bond order enables chemists to predict the properties and reactivity of compounds. For instance, in the carbon dioxide molecule (CO2), the carbon-oxygen double bond indicates a bond order of 2, implying a stronger bond than a single bond.
Delving into this topic, we will explore the methods for calculating bond order, their significance in understanding chemical behavior, and the historical development of these concepts.
How to Determine Bond Order from Structure
Determining bond order from structure is a crucial aspect of understanding chemical bonding. It involves analyzing various factors:
- Atomic number
- Electronegativity
- Hybridization
- Resonance
- Formal charge
- Oxidation state
- Molecular orbital theory
- Valence bond theory
- X-ray crystallography
- Spectroscopy
These aspects provide insights into the electronic structure, geometry, and bonding behavior of molecules. Understanding bond order enables chemists to predict properties, reactivity, and design new materials with desired characteristics.
Atomic number
Atomic number plays a fundamental role in determining bond order from structure. It represents the number of protons in an atom’s nucleus and defines an element’s identity and chemical properties.
- Proton Count: The atomic number directly corresponds to the number of protons in the nucleus, determining an element’s position on the periodic table.
- Nuclear Charge: The atomic number determines the net positive charge of the nucleus, which attracts electrons and influences bond formation.
- Electronegativity: Atomic number influences electronegativity, the ability of an atom to attract electrons. This affects bond polarity and, consequently, bond order.
- Atomic Radius: The atomic number influences the size of an atom, which can impact bond lengths and strengths.
In summary, atomic number provides crucial information about an atom’s nuclear charge, electronegativity, and size, all of which are essential factors in determining bond order from structure.
Electronegativity
In the context of determining bond order from structure, electronegativity plays a crucial role. It represents the ability of an atom to attract electrons towards itself within a chemical bond.
- Atomic Electronegativity: Electronegativity is an intrinsic property of an atom, influenced by its atomic number and size.
- Bond Electronegativity: Electronegativity can also be considered at the bond level, representing the average electronegativity of the bonded atoms.
- Electronegativity Difference: The difference in electronegativity between bonded atoms influences bond polarity and bond order.
- Electronegativity and Resonance: Electronegativity can affect the extent of resonance in a molecule, which in turn influences bond order.
Understanding electronegativity provides insights into the distribution of electrons within a molecule, helping determine bond order and predict molecular properties.
Hybridization
Hybridization plays a vital role in determining bond order from structure by explaining the bonding behavior of atoms. It involves the mixing of atomic orbitals to form new hybrid orbitals with different shapes and energies.
- Atomic Orbitals: These are the orbitals present in isolated atoms before hybridization occurs.
- Hybrid Orbitals: These are the new orbitals formed by combining atomic orbitals, having different shapes and energies than the original atomic orbitals.
- Types of Hybridization: The type of hybridization depends on the number and type of atomic orbitals involved in the hybridization process.
- Bonding in Hybridized Orbitals: Hybridized orbitals overlap to form sigma and pi bonds, determining the geometry and bond order of the molecule.
By understanding hybridization, chemists can predict the molecular geometry, bond order, and properties of compounds, providing valuable insights into their chemical behavior and reactivity.
Resonance
Resonance plays a significant role in determining bond order from structure, providing a more accurate representation of the electronic structure and bonding in certain molecules. It involves the concept of delocalization of electrons over multiple atomic centers, leading to the formation of resonance structures.
- Equivalent Resonance Structures: Resonance structures are hypothetical Lewis structures that depict the various possible electron distributions within a molecule, with the actual structure being a hybrid of these resonance forms.
- Delocalization of Electrons: Resonance involves the delocalization of electrons over multiple atoms, resulting in a more stable and lower-energy state for the molecule.
- Implications for Bond Order: Resonance structures can have different bond orders compared to the original Lewis structure, as the electron distribution is spread out over multiple bonds.
- Aromatic Compounds: Resonance is particularly important in aromatic compounds, where the delocalization of electrons over a ring of atoms leads to increased stability and unique chemical properties.
Considering resonance when determining bond order provides a more accurate understanding of the electronic structure and bonding in molecules, allowing for better predictions of their chemical behavior and reactivity.
Formal charge
Formal charge is a crucial concept in determining bond order from structure. It represents the hypothetical charge assigned to an atom in a molecule, assuming all bonds are purely ionic. Understanding formal charge provides insights into the electron distribution and bonding behavior within a molecule.
Formal charge affects bond order as it helps determine the most likely Lewis structure for a molecule. By calculating the formal charges of different atoms in a molecule, chemists can identify the most stable resonance structure, which corresponds to the lowest overall formal charge. This, in turn, helps determine the bond order, as the formal charge distribution influences the electron density and bond strength.
For example, in the carbonate ion (CO32-), the formal charge on each oxygen atom is -1, while the formal charge on the carbon atom is +2. This distribution indicates that the carbon atom has a higher electron density and stronger bonds to the oxygen atoms, consistent with the observed double bonds in the Lewis structure. Understanding formal charge thus enables chemists to rationalize and predict bond order based on the distribution of electrons within a molecule.
In summary, formal charge plays a significant role in determining bond order from structure by providing insights into the electron distribution and bonding behavior within a molecule. It helps identify the most stable Lewis structure and rationalize the observed bond orders, aiding in the understanding and prediction of molecular properties and reactivity.
Oxidation state
Oxidation state, a crucial aspect in determining bond order from structure, provides insights into the electron distribution and bonding behavior within a molecule. It represents the hypothetical charge assigned to an atom, assuming all bonds are purely ionic.
- Assigning Oxidation States: Oxidation states can be assigned to atoms in a molecule based on specific rules, considering the electronegativity of atoms and the distribution of electrons.
- Predicting Bond Order: Oxidation states can help predict bond order by indicating the electron density around each atom. Higher oxidation states generally correspond to lower electron density and stronger bonds.
- Resonance and Oxidation States: In molecules with resonance structures, oxidation states can vary across different resonance forms. Understanding these variations is essential for accurately determining bond order.
- Exceptional Cases: Transition metal complexes often exhibit variable oxidation states, which can affect their electronic structure and bonding properties.
Overall, considering oxidation states provides valuable insights into the electronic structure and bonding behavior of molecules, aiding in the determination of bond order from structure. It helps rationalize the observed bond orders and predict the reactivity and properties of compounds.
Molecular orbital theory
In the realm of “how to determine bond order from structure,” molecular orbital (MO) theory stands as an indispensable tool. It provides a comprehensive framework for understanding the electronic structure and bonding behavior of molecules, offering valuable insights for determining bond order.
- Linear combination of atomic orbitals (LCAO): MO theory employs LCAO, combining atomic orbitals to form molecular orbitals, which are spread over the entire molecule.
- Molecular orbital diagram: This diagram depicts the energy levels and shapes of molecular orbitals, aiding in the visualization of electron distribution and bond formation.
- Bonding and antibonding orbitals: MOs are classified as bonding (lower energy, electron density concentrated between nuclei) or antibonding (higher energy, electron density concentrated away from nuclei), influencing bond order.
- Electron configuration and bond order: The number and arrangement of electrons in MOs determine the bond order, providing a quantitative measure of bond strength.
By unraveling the intricate details of molecular electronic structure, MO theory empowers chemists to accurately determine bond order from structure, enabling predictions of molecular properties, reactivity, and behavior.
Valence bond theory
Valence bond theory (VBT) plays a central role in understanding “how to determine bond order from structure”. VBT focuses on the interactions between atomic orbitals to form covalent bonds, providing a qualitative description of bond formation and properties.
A key aspect of VBT is the concept of hybridization, where atomic orbitals combine to form new hybrid orbitals with specific shapes and orientations. These hybrid orbitals then overlap with orbitals from other atoms to form sigma and pi bonds. The type of hybridization and the number of overlapping orbitals determine the bond order and, consequently, the strength and properties of the bond.
For example, in the methane molecule (CH4), the carbon atom undergoes sp3 hybridization, resulting in four equivalent hybrid orbitals. These hybrid orbitals overlap with the 1s orbitals of four hydrogen atoms, forming four equivalent sigma bonds with a bond order of 1. This understanding, based on VBT, allows us to determine the tetrahedral geometry and predict the properties of methane.
VBT not only helps determine bond order but also provides insights into molecular stability, reactivity, and magnetic properties. By analyzing the overlap and interactions between atomic orbitals, chemists can gain a deeper understanding of the electronic structure and behavior of molecules.
X-ray crystallography
X-ray crystallography is a powerful technique that has revolutionized the field of structural chemistry. It involves directing X-rays at a crystal sample and analyzing the resulting diffraction patterns to determine the arrangement of atoms within the crystal. This information can be used to determine bond lengths, bond angles, and molecular geometry, all of which are essential for understanding the bonding and properties of materials.
X-ray crystallography is a critical component of “how to determine bond order from structure”. By determining the bond lengths and angles, we can gain insights into the strength and type of chemical bonds present in a molecule. This information can be used to predict the physical and chemical properties of the molecule, such as its reactivity, stability, and solubility. For example, X-ray crystallography was used to determine the structure of DNA, which revealed the double helix structure and provided a foundation for understanding genetics.
X-ray crystallography is a versatile technique that can be used to study a wide variety of materials, including organic and inorganic compounds, metals, and alloys. It has applications in fields such as drug discovery, materials science, and catalysis. By understanding the structure of materials, scientists can design new materials with improved properties and tailor materials for specific applications.
In summary, X-ray crystallography is a powerful tool that allows scientists to determine the structure of materials at the atomic level. This information is essential for understanding the bonding and properties of materials and has a wide range of applications in various scientific disciplines.
Spectroscopy
Spectroscopy is a critical component of “how to determine bond order from structure” because it allows scientists to analyze the electromagnetic radiation absorbed or emitted by a molecule and relate it to its structure and bonding. By studying the characteristic spectral lines or bands associated with different molecular vibrations, rotations, and electronic transitions, spectroscopists can gain valuable insights into the molecular structure, including bond lengths, bond angles, and the presence of functional groups.
One of the most common spectroscopic techniques used to determine bond order is infrared (IR) spectroscopy. IR spectroscopy measures the absorption of infrared radiation by a molecule, which corresponds to the vibrational modes of the bonds. The frequency of the absorbed radiation is directly related to the strength of the bond, with stronger bonds absorbing at higher frequencies. By analyzing the IR spectrum of a molecule, chemists can identify the different types of bonds present and estimate their bond orders.
Another important spectroscopic technique is nuclear magnetic resonance (NMR) spectroscopy. NMR spectroscopy measures the magnetic properties of atomic nuclei, which are sensitive to their chemical environment. By analyzing the NMR spectrum of a molecule, chemists can determine the connectivity of atoms and identify different functional groups. This information can be used to deduce the molecular structure and, in some cases, the bond orders.
In summary, spectroscopy plays a vital role in “how to determine bond order from structure” by providing detailed information about the molecular structure and bonding. Spectroscopic techniques, such as IR and NMR spectroscopy, allow scientists to analyze the electromagnetic radiation absorbed or emitted by molecules and relate it to their structure and properties. This understanding is essential for various fields of science, including chemistry, biology, and materials science, and has practical applications in drug discovery, materials design, and quality control.
Frequently Asked Questions on Determining Bond Order from Structure
This section addresses common questions and misconceptions surrounding the topic of “how to determine bond order from structure.” The following FAQs provide concise answers to guide your understanding of this important concept.
Question 1: What is the significance of bond order in understanding molecular properties?
Answer: Bond order provides insights into the strength, length, and polarity of chemical bonds. It helps explain molecular properties such as stability, reactivity, and magnetic behavior.
Question 2: How can I determine bond order using electronegativity?
Answer: Electronegativity differences between bonded atoms influence bond order. Higher electronegativity differences lead to more polar bonds and lower bond orders.
Question 3: What role does hybridization play in bond order determination?
Answer: Hybridization involves the mixing of atomic orbitals to form new hybrid orbitals. Different types of hybridization result in different bond orders and molecular geometries.
Question 4: How does resonance affect bond order?
Answer: Resonance involves the delocalization of electrons over multiple atoms. It can lead to non-integer bond orders and alter the overall electronic structure of the molecule.
Question 5: What experimental techniques can be used to determine bond order?
Answer: Techniques such as X-ray crystallography and spectroscopy provide valuable information about bond lengths, bond angles, and molecular vibrations, which can be used to infer bond order.
Question 6: Why is it important to consider multiple factors when determining bond order?
Answer: Bond order is influenced by various factors such as atomic number, electronegativity, hybridization, and resonance. Considering all these factors provides a more accurate and comprehensive understanding of bond order.
In summary, these FAQs have highlighted the key aspects and considerations involved in determining bond order from structure. Understanding bond order is crucial for predicting molecular properties, reactivity, and behavior, which are essential concepts in chemistry.
In the next section, we will delve deeper into the applications of bond order in various fields of science and explore how it aids in the design and development of new materials and pharmaceuticals.
Tips for Determining Bond Order from Structure
This section provides practical tips to enhance your understanding and accuracy when determining bond order from structure.
Tip 1: Consider Atomic Number and Electronegativity: Atomic number and electronegativity influence bond strength and polarity, impacting bond order.
Tip 2: Analyze Hybridization: Hybridization describes the mixing of atomic orbitals, affecting bond geometry and bond order.
Tip 3: Examine Resonance Structures: Resonance involves delocalized electrons, leading to non-integer bond orders and altered electronic structures.
Tip 4: Utilize Molecular Orbital Theory: MO theory provides a framework for understanding electron distribution and bond formation, aiding in bond order determination.
Tip 5: Apply Valence Bond Theory: VBT focuses on atomic orbital interactions and hybridization, offering insights into bond order and molecular stability.
Tip 6: Leverage X-ray Crystallography: This technique reveals bond lengths and angles, enabling the determination of bond order.
Tip 7: Employ Spectroscopy: Spectroscopic techniques, such as IR and NMR spectroscopy, provide information about molecular vibrations and connectivity, aiding in bond order analysis.
Understanding and applying these tips will empower you to accurately determine bond order and gain a deeper comprehension of molecular structures and properties.
In the concluding section, we will explore the significance of determining bond order in diverse fields, including chemistry, biology, and materials science, highlighting its role in advancing scientific research and technological applications.
Conclusion
This comprehensive exploration of “how to determine bond order from structure” has unveiled the fundamental principles and techniques that empower chemists to unravel the intricate details of molecular bonding. Key concepts, such as electronegativity, hybridization, resonance, and molecular orbital theory, provide a solid foundation for understanding and predicting bond order.
The interconnectedness of these concepts highlights the importance of considering various factors when determining bond order. For instance, electronegativity influences the polarity of bonds, which in turn affects hybridization and resonance. Understanding these relationships enables chemists to accurately predict molecular properties and design new materials with tailored characteristics.
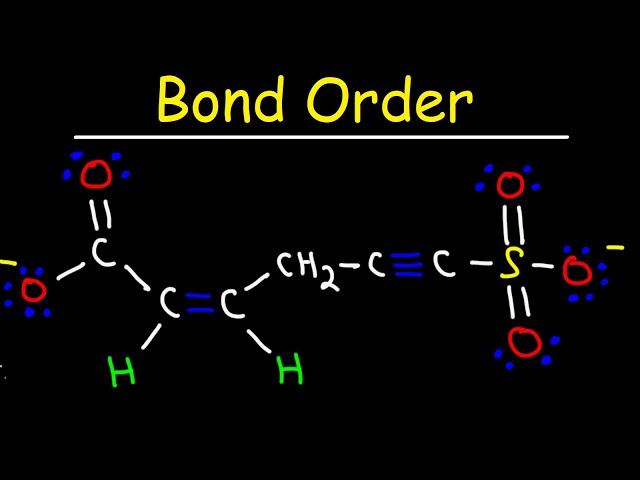