Bond Order Calculation for Nitrogen Oxides – A Comprehensive Guide
Determining bond order involves understanding the number of chemical bonds between atoms in a molecule. In the case of nitrogen oxides, calculating bond order is crucial for comprehending their chemical properties and reactivity. This article unveils a step-by-step approach to calculating bond order in nitrogen oxides, providing insights into their molecular structures and applications.
Bond order plays a significant role in predicting the stability, reactivity, and physical properties of nitrogen oxides. By understanding bond order, researchers and scientists can gain valuable information about the behavior and potential uses of these compounds in various fields, including atmospheric chemistry, combustion processes, and fertilizer production.
How to Calculate Bond Order of NO
Understanding bond order is crucial for comprehending the chemical properties and reactivity of nitrogen oxides.
- Number of atomic orbitals involved
- Types of atomic orbitals involved
- Molecular orbital theory
- Electron configuration
- Bond length
- Bond energy
- Magnetic properties
- Spectroscopic properties
- Thermodynamic stability
- Reaction mechanisms
These aspects provide valuable insights into the behavior and potential uses of nitrogen oxides in various fields, including atmospheric chemistry, combustion processes, and fertilizer production.
Number of Atomic Orbitals Involved
Determining the number of atomic orbitals involved is a crucial step in calculating bond order, as it provides insights into the nature and strength of the chemical bond between atoms. In the context of nitrogen oxides, the number of atomic orbitals involved directly affects the bond order and, consequently, the overall stability and reactivity of the molecule.
- Atomic Orbital Overlap
The extent to which atomic orbitals overlap determines the strength and type of covalent bond formed. Greater overlap leads to stronger bonds and higher bond orders. - Types of Atomic Orbitals
The type of atomic orbitals involved (e.g., s, p, d) influences the shape and directionality of the molecular orbital formed. This affects the bond order and the overall molecular geometry. - Hybridization
Hybridization of atomic orbitals can lead to the formation of new hybrid orbitals with different shapes and energies. This can alter the number of atomic orbitals available for bonding and impact the bond order. - Unpaired Electrons
The presence of unpaired electrons in atomic orbitals can influence bond order by promoting electron pairing and the formation of stronger bonds.
Understanding the number and types of atomic orbitals involved in bond formation is essential for accurately calculating bond order and predicting the properties and behavior of nitrogen oxides.
Types of Atomic Orbitals Involved
In calculating bond order, the types of atomic orbitals involved play a crucial role in determining the strength and nature of the chemical bond. Different atomic orbitals have distinct shapes and orientations, which influence the overlap and interaction between them, ultimately affecting the bond order.
- s Orbitals
s orbitals are spherical in shape and have no directional properties. They are involved in sigma () bond formation, which is the strongest type of covalent bond. - p Orbitals
p orbitals have a dumbbell shape with two lobes. They can overlap laterally to form pi () bonds, which are weaker than sigma bonds. Multiple bonds can form between two atoms, resulting in higher bond orders. - d Orbitals
d orbitals have more complex shapes and can participate in both sigma and pi bond formation. They are commonly found in transition metal complexes and can contribute to the formation of multiple bonds. - Hybridization
Atomic orbitals can hybridize to form new hybrid orbitals with different shapes and energies. Hybridization can alter the number and type of atomic orbitals available for bonding, influencing the bond order and molecular geometry.
Understanding the types of atomic orbitals involved in bond formation is crucial for accurately calculating bond order and predicting the properties and behavior of nitrogen oxides and other molecules.
Molecular Orbital Theory
Molecular orbital theory (MOT) is a fundamental concept in quantum chemistry that describes the electronic structure of molecules. It provides a mathematical framework for understanding the bonding, properties, and behavior of molecules. MOT is crucial for calculating bond order, as it offers a detailed account of the molecular orbitals formed by the interaction of atomic orbitals.
In MOT, electrons occupy molecular orbitals, which are the result of the combination of atomic orbitals. The type and energy of the molecular orbitals depend on the symmetry and overlap of the atomic orbitals involved. By applying MOT, chemists can determine the number and type of chemical bonds between atoms, which is reflected in the bond order.
For example, in the nitrogen monoxide (NO) molecule, MOT explains the formation of a triple bond between the nitrogen and oxygen atoms. The atomic orbitals involved are the nitrogen 2p orbitals and the oxygen 2p orbitals. These orbitals overlap to form three molecular orbitals: a bonding sigma orbital (), a bonding pi orbital (), and an antibonding pi orbital (*). The two bonding orbitals are filled with electrons, resulting in a bond order of three, which corresponds to the triple bond observed in NO.
Understanding the connection between MOT and bond order calculations is essential for comprehending the electronic structure and properties of molecules. MOT provides a powerful tool for predicting bond strengths, molecular geometries, and chemical reactivity, which are crucial for various fields, including materials science, catalysis, and drug design.
Electron Configuration
Electron configuration plays a critical role in determining the bond order of nitrogen monoxide (NO). It describes the distribution of electrons in atomic orbitals, providing insights into the chemical bonding and properties of the molecule.
- Atomic Orbitals
Atomic orbitals are the specific regions around the nucleus where electrons are most likely to be found. The type and number of atomic orbitals involved in bonding determine the bond order. - aufbau principle
The aufbau principle states that electrons fill atomic orbitals in order of increasing energy. This principle helps determine the electron configuration of atoms and, subsequently, the bond order of molecules. - Pauli Exclusion Principle
The Pauli exclusion principle states that no two electrons can occupy the same quantum state. This principle restricts the number of electrons that can occupy each atomic orbital and influences the bond order. - Hund’s Rule
Hund’s rule states that electrons occupy degenerate orbitals (orbitals with the same energy) with parallel spins before pairing. This rule affects the electron configuration and, consequently, the bond order of molecules.
Understanding electron configuration is essential for accurately calculating bond order and predicting the properties and behavior of nitrogen monoxide. By considering the distribution of electrons in atomic orbitals, chemists can gain valuable insights into the nature of the chemical bond between nitrogen and oxygen.
Bond length
Bond length is a critical component of calculating bond order in nitrogen monoxide (NO). It refers to the distance between the nuclei of the bonded atoms and is inversely proportional to bond order. A shorter bond length typically corresponds to a higher bond order, indicating a stronger bond between the atoms.
The bond length in NO can be determined experimentally using techniques such as X-ray crystallography or microwave spectroscopy. The bond length provides valuable insights into the nature of the chemical bond between nitrogen and oxygen. A shorter bond length suggests a stronger bond, which is consistent with the triple bond character of NO.
Understanding the relationship between bond length and bond order is crucial for predicting the properties and behavior of NO. For example, the short bond length in NO contributes to its high bond strength and stability. This understanding finds practical applications in various fields, including atmospheric chemistry, combustion processes, and fertilizer production.
In summary, bond length is a critical parameter in calculating bond order, providing insights into the strength and nature of the chemical bond. The inverse relationship between bond length and bond order allows chemists to predict and understand the properties and behavior of molecules like nitrogen monoxide.
Bond energy
Bond energy is a crucial aspect of calculating bond order in nitrogen monoxide (NO) and provides valuable insights into the strength and stability of the chemical bond. It refers to the energy required to break a bond between two atoms and is directly related to bond order. A higher bond order typically corresponds to a higher bond energy, indicating a stronger bond.
Bond energy can be determined experimentally using techniques such as calorimetry or spectroscopy. The bond energy of NO is relatively high, reflecting the triple bond character of the molecule. The strong bond energy contributes to the stability of NO and its resistance to dissociation.
Understanding the connection between bond energy and bond order is essential for predicting the behavior of NO in various chemical reactions and processes. For example, the high bond energy of NO makes it a relatively inert molecule, less likely to undergo bond breaking or rearrangement. This understanding finds practical applications in fields such as atmospheric chemistry, combustion processes, and fertilizer production, where NO plays a significant role.
In summary, bond energy is a critical component in calculating bond order, providing insights into the strength and stability of the chemical bond. The relationship between bond energy and bond order allows chemists to predict and understand the properties and behavior of molecules like nitrogen monoxide.
Magnetic properties
Magnetic properties play a significant role in understanding the electronic structure and bonding of nitrogen monoxide (NO). These properties arise from the unpaired electrons in the molecule and provide valuable insights into its chemical behavior and reactivity.
- Paramagnetism
NO is a paramagnetic molecule due to the presence of one unpaired electron in its molecular orbital. This unpaired electron contributes to the magnetic moment of the molecule, allowing it to be attracted to a magnetic field. - Magnetic Susceptibility
The magnetic susceptibility of NO is a measure of its response to an applied magnetic field. The positive magnetic susceptibility of NO indicates that it is attracted to magnetic fields. - Electron Spin Resonance (ESR)
ESR spectroscopy can be used to study the magnetic properties of NO. ESR measures the absorption of microwave radiation by unpaired electrons, providing information about their number, environment, and interactions. - Magnetic Moment
The magnetic moment of NO is related to the number of unpaired electrons and their spin orientations. The magnetic moment can be used to determine the electronic configuration and bonding of the molecule.
Understanding the magnetic properties of NO contributes to the overall comprehension of its electronic structure and chemical bonding. These properties provide valuable insights into the molecule’s reactivity, stability, and behavior in various chemical processes.
Spectroscopic properties
Spectroscopic properties provide valuable insights into the electronic structure and bonding of molecules, including nitrogen monoxide (NO). By analyzing the interaction of electromagnetic radiation with molecules, we can obtain information about their energy levels, vibrational modes, and electronic transitions.
- Absorption Spectroscopy
Absorption spectroscopy measures the absorption of light by molecules, providing information about the energy levels and transitions between them. This technique can be used to identify and quantify NO in various samples. - Emission Spectroscopy
Emission spectroscopy analyzes the emission of light by molecules when they return to a lower energy state. This technique can provide insights into the excited states and electronic structure of NO. - Vibrational Spectroscopy
Vibrational spectroscopy investigates the vibrational modes of molecules, providing information about their bond strengths and molecular geometry. This technique can help determine the vibrational frequencies and functional groups associated with NO. - Mass Spectrometry
Mass spectrometry separates and analyzes ions based on their mass-to-charge ratio. This technique can be used to identify and quantify NO in complex mixtures, such as atmospheric samples.
Understanding the spectroscopic properties of NO is crucial for characterizing its electronic structure, bonding, and behavior in various chemical processes. These spectroscopic techniques provide complementary information that helps researchers gain a comprehensive understanding of this important molecule.
Thermodynamic Stability
Thermodynamic stability plays a crucial role in understanding the behavior and properties of nitrogen oxides (NOx), influencing their bond order and reactivity. It refers to the tendency of a molecule to maintain its structural integrity and resist decomposition under specific conditions.
- Free Energy
Free energy is a measure of the spontaneity and stability of a chemical reaction. Lower free energy indicates a more stable state. Calculating bond order helps estimate the free energy change associated with the formation or breaking of chemical bonds, providing insights into the thermodynamic stability of NOx.
- Bond Strength
Bond strength is directly related to bond order, with higher bond orders typically indicating stronger bonds. Thermodynamically stable molecules possess strong bonds that resist breaking, leading to a lower free energy and increased stability.
- Reaction Equilibria
In chemical reactions, the position of equilibrium is influenced by the thermodynamic stability of the reactants and products. By calculating bond orders, chemists can estimate the relative stability of different species and predict the direction and extent of chemical reactions.
- Applications in NOx Control
Understanding the thermodynamic stability of NOx is crucial for developing effective strategies to control NOx emissions. By manipulating bond orders through catalytic processes or additives, engineers can design systems that promote the formation of more stable and less harmful NOx compounds.
In summary, thermodynamic stability is an essential aspect of understanding bond order calculations for NOx. It provides valuable insights into the stability, reactivity, and behavior of these compounds, enabling researchers and engineers to optimize processes and mitigate environmental concerns related to NOx emissions.
Reaction mechanisms
Understanding reaction mechanisms is critical for calculating bond order accurately. Reaction mechanisms describe the step-by-step pathway of a chemical reaction, including the breaking and formation of bonds. By analyzing the reaction mechanism, we can identify the intermediate species and transition states involved, which are crucial for determining the bond order at each stage of the reaction.
For example, in the reaction of nitrogen monoxide (NO) with oxygen (O2) to form nitrogen dioxide (NO2), the reaction proceeds through a two-step mechanism. In the first step, NO reacts with O2 to form an intermediate species, NO3. In the second step, NO3 reacts with another NO molecule to form NO2. By calculating the bond order of NO, O2, NO3, and NO2 at each step of the mechanism, we can gain insights into the stability and reactivity of these species and predict the overall rate and selectivity of the reaction.
Furthermore, understanding reaction mechanisms allows us to design and optimize catalytic processes for various industrial applications. By manipulating the reaction conditions and using appropriate catalysts, we can control the bond order of the reactants and products, thereby improving the efficiency and selectivity of the desired chemical reactions. This knowledge is essential for developing sustainable and environmentally friendly chemical processes.
Frequently Asked Questions about Bond Order Calculation for Nitrogen Oxides
This FAQ section addresses common questions and clarifications regarding the calculation of bond order in nitrogen oxides (NOx).
Question 1: What is bond order, and why is it important?
Answer: Bond order describes the number of chemical bonds between two atoms in a molecule. It provides insights into the strength and stability of the bond, influencing the molecule’s overall properties and reactivity.
Question 2: How do I calculate the bond order of nitrogen monoxide (NO)?
Answer: The bond order of NO is 2.5, indicating a triple bond between nitrogen and oxygen. This can be determined using molecular orbital theory, considering the number and overlap of atomic orbitals involved in the bond.
Question 3: What factors affect the bond order of NOx compounds?
Answer: Factors such as the number of valence electrons, hybridization of atomic orbitals, and the presence of resonance structures can influence the bond order of NOx compounds.
Question 4: How does bond order relate to bond length and bond energy?
Answer: Bond order is inversely proportional to bond length and directly proportional to bond energy. Shorter bond lengths and higher bond energies indicate higher bond orders, reflecting stronger bonds.
Question 5: What are the applications of bond order calculations in NOx chemistry?
Answer: Bond order calculations help predict the stability, reactivity, and spectroscopic properties of NOx compounds. This knowledge is valuable in fields such as atmospheric chemistry, combustion processes, and fertilizer production.
Question 6: How can I use bond order to better understand the behavior of NOx in environmental systems?
Answer: By understanding the bond order of NOx compounds, scientists can gain insights into their formation, transport, and deposition in the environment. This knowledge aids in developing strategies to mitigate NOx pollution and protect air quality.
These FAQs provide a concise overview of key concepts related to bond order calculations for nitrogen oxides. For a more in-depth exploration of this topic, refer to the comprehensive article sections that follow.
Transition to the next article section: Understanding bond order is essential for comprehending the chemical properties and behavior of nitrogen oxides. The following sections will delve into the theoretical foundations and practical applications of bond order calculations, providing a deeper understanding of these important compounds.
Tips for Accurate Bond Order Calculation in Nitrogen Oxides
Precise bond order calculations are crucial for understanding the behavior and properties of nitrogen oxides. Here are several practical tips to ensure accurate results:
Tip 1: Determine Valence Electrons
Identify the total number of valence electrons involved in the bonding between nitrogen and oxygen atoms.
Tip 2: Construct Molecular Orbital Diagram
Depict the molecular orbitals formed by the overlap of atomic orbitals, considering their symmetry and energy levels.
Tip 3: Count Bonding Electrons
Determine the number of electrons occupying the bonding molecular orbitals, which contribute to the bond order.
Tip 4: Subtract Antibonding Electrons
Identify the antibonding molecular orbitals and subtract the number of electrons occupying them from the bonding electrons.
Tip 5: Divide by Two
The bond order is obtained by dividing the result of step 4 by two.
Tip 6: Consider Resonance Structures
If resonance structures exist, calculate the bond order for each structure and take the average.
Tip 7: Utilize Computational Chemistry Tools
Employ computational chemistry software to assist with complex bond order calculations and visualize molecular orbitals.
Tip 8: Validate Results Experimentally
Compare the calculated bond order with experimental data, such as bond length or vibrational frequencies, to ensure accuracy.
By following these tips, researchers can ensure the accuracy and reliability of their bond order calculations for nitrogen oxides, enabling a deeper understanding of their chemical properties and behavior.
Transition to the conclusion: Accurate bond order calculations provide valuable insights into the stability, reactivity, and spectroscopic properties of nitrogen oxides. These insights are essential for advancing research in atmospheric chemistry, combustion processes, and fertilizer production.
Conclusion
This article has provided a comprehensive guide on calculating bond order in nitrogen oxides, a critical aspect for understanding their chemical properties and behavior. We have explored the theoretical foundations, practical applications, and tips for accurate calculations.
Key points to remember include:
- Bond order reflects the strength and stability of the bond between nitrogen and oxygen atoms.
- Molecular orbital theory provides a framework for understanding the formation and properties of molecular orbitals, which contribute to bond order.
- Accurate bond order calculations enable researchers to predict the stability, reactivity, and spectroscopic properties of nitrogen oxides, aiding in various fields such as atmospheric chemistry and combustion processes.
The ability to calculate bond order empowers scientists to gain deeper insights into the behavior of nitrogen oxides in environmental and industrial settings. By harnessing this knowledge, we can develop more effective strategies for pollution control and optimize processes involving nitrogen oxides.
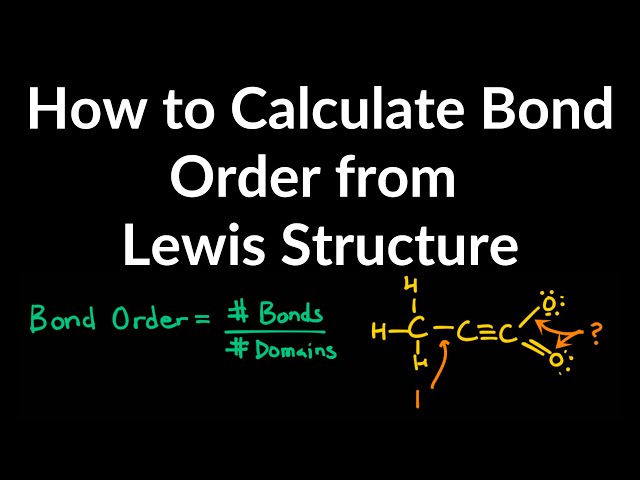