Bond order is a crucial concept in chemistry, representing the strength and type of chemical bond between atoms. Finding examples of bond order calculations is essential for understanding its practical applications.
Bond order calculations help determine molecular properties, such as stability, reactivity, and magnetic behavior. Historically, Linus Pauling developed the concept of bond order in the 1930s, providing a fundamental framework for understanding chemical bonding.
This article will explore various methods to find bond order examples, including molecular orbital theory, valence bond theory, and experimental techniques like X-ray crystallography and nuclear magnetic resonance (NMR) spectroscopy.
How to Find Bond Order Examples
Understanding the methods to find bond order examples is crucial for comprehending the nature of chemical bonding and molecular properties.
- Molecular Orbital Theory
- Valence Bond Theory
- X-ray Crystallography
- Nuclear Magnetic Resonance (NMR) Spectroscopy
- Quantum Chemistry Calculations
- Experimental Measurements
- Periodic Trends
- Resonance Structures
- Hybridization
These aspects provide a comprehensive approach to finding bond order examples, encompassing theoretical frameworks, experimental techniques, and fundamental chemical concepts. Understanding these aspects deepens our knowledge of chemical bonding and its impact on molecular structure and reactivity.
Molecular Orbital Theory
Molecular Orbital Theory (MOT) provides a powerful framework for understanding and predicting the electronic structure of molecules, including the bond order between atoms. It describes molecular orbitals as mathematical functions that represent the wave-like behavior of electrons within a molecule. By analyzing these molecular orbitals, we can determine the number and type of chemical bonds present in a molecule, thus providing a direct connection to bond order determination.
MOT is a critical component of “how to find bond order examples” because it offers a theoretical basis for calculating and predicting bond orders. It allows us to visualize the molecular orbitals involved in bonding and understand how they contribute to the overall bond strength and stability. For instance, in the case of the hydrogen molecule (H2), MOT predicts a bond order of 1, indicating a single covalent bond between the two hydrogen atoms. This aligns with experimental observations and the observed stability of the H2 molecule.
The practical applications of understanding the connection between MOT and bond order extend to various fields of chemistry. In inorganic chemistry, it helps predict and explain the bonding and properties of coordination complexes. In organic chemistry, it aids in understanding the reactivity and selectivity of organic molecules, guiding the design of new drugs and materials. Furthermore, MOT provides insights into the electronic and magnetic properties of materials, with applications in fields such as solid-state physics and materials science.
Valence Bond Theory
Valence Bond Theory (VBT) is another valuable tool for understanding and finding bond order examples. VBT focuses on the interactions between atomic orbitals to form molecular orbitals and, subsequently, chemical bonds.
- Hybrid Orbitals
VBT explains how atomic orbitals can hybridize to form new orbitals with different shapes and energies. These hybrid orbitals then overlap to form sigma and pi bonds, contributing to the overall bond order of the molecule.
- Resonance Structures
VBT allows us to describe resonance structures, which are different Lewis structures that contribute to the overall bonding of a molecule. By considering the resonance structures and their relative contributions, we can determine the most accurate bond order for the molecule.
- Bond Order and Properties
VBT helps us understand the relationship between bond order and molecular properties. For instance, higher bond orders typically correspond to shorter bond lengths and stronger bonds, providing insights into the stability and reactivity of molecules.
- Applications in Chemistry
VBT has broad applications in chemistry, including predicting the structures and properties of molecules, rationalizing reaction mechanisms, and understanding the bonding in complex systems such as coordination compounds and organometallic species.
By considering the principles of VBT and its implications for bond order determination, we gain a deeper understanding of the electronic structure and chemical behavior of molecules.
X-ray Crystallography
X-ray crystallography plays a crucial role in “how to find bond order examples” by providing experimental data that allows scientists to determine the precise arrangement of atoms and their bonding within a crystal lattice. X-rays, a form of electromagnetic radiation, are directed at a crystal, and the resulting diffraction pattern is analyzed to reveal the electron density distribution within the crystal.
This information is vital for bond order determination because it allows scientists to directly visualize the atomic orbitals involved in bonding and measure the distance between atoms. By analyzing the electron density distribution, scientists can determine the type and strength of chemical bonds present, thus providing a direct and accurate method for finding bond order examples.
Real-life examples of X-ray crystallography’s application in bond order determination include the study of metal-organic frameworks (MOFs), complex materials with well-defined structures and potential applications in gas storage and catalysis. X-ray crystallography has also been used to determine the bond order in high-temperature superconductors, materials that exhibit zero electrical resistance at certain temperatures. These examples showcase the broad applicability of X-ray crystallography in understanding chemical bonding and materials properties.
The practical applications of understanding bond order through X-ray crystallography extend to various fields of science and technology. In materials science, it aids in the design and development of new materials with tailored properties. In biology, it contributes to the understanding of protein structure and function, providing insights into enzyme mechanisms and drug design. Furthermore, X-ray crystallography is essential in the pharmaceutical industry for determining the molecular structure of drug candidates and understanding their interactions with biological targets.
Nuclear Magnetic Resonance (NMR) Spectroscopy
Nuclear Magnetic Resonance (NMR) Spectroscopy is a powerful analytical tool that provides valuable insights into the structure and dynamics of molecules. Its connection to “how to find bond order examples” lies in the ability of NMR to probe the electronic environment of atoms within a molecule.
NMR spectroscopy exploits the magnetic properties of certain atomic nuclei, such as 1H and 13C, to determine their chemical environment. By analyzing the chemical shifts and coupling constants observed in NMR spectra, scientists can deduce the types of atoms bonded to a particular atom and the geometry of the surrounding molecular framework. This information directly contributes to the determination of bond orders, as bond order is influenced by the number and type of neighboring atoms.
For instance, in the case of ethene (C2H4), 1H NMR spectroscopy can distinguish between the chemically distinct hydrogen atoms (Ha and Hb) and reveal their coupling patterns. The observed coupling constants provide information about the dihedral angle between the Ha and Hb protons, which is directly related to the C=C bond order. Higher bond orders typically result in smaller dihedral angles and larger coupling constants.
The practical applications of understanding bond order through NMR spectroscopy extend to various fields of chemistry. In organic chemistry, it aids in the structure elucidation of complex organic molecules, including natural products and pharmaceuticals. In inorganic chemistry, NMR spectroscopy helps determine the coordination environment of metal ions in coordination complexes. Furthermore, NMR spectroscopy is essential in materials science for characterizing the structure and dynamics of polymers, ceramics, and other materials.
Quantum Chemistry Calculations
Quantum chemistry calculations, grounded in the principles of quantum mechanics, provide a powerful tool for “how to find bond order examples”. These calculations enable us to determine the electronic structure of molecules, including the nature and strength of chemical bonds.
- Hartree-Fock Theory
The Hartree-Fock method is a self-consistent field approach that approximates the wavefunction of a molecule as a product of one-electron wavefunctions. By solving the Hartree-Fock equations, we obtain molecular orbitals and their corresponding energies, which can be used to determine bond orders.
- Density Functional Theory (DFT)
DFT is a more sophisticated approach that considers the electron density of a molecule rather than the wavefunction. DFT calculations provide accurate bond orders and insights into the electronic properties of molecules.
- Molecular Orbital Theory
Quantum chemistry calculations based on molecular orbital theory allow us to visualize the molecular orbitals involved in bonding and analyze their interactions. This provides a qualitative understanding of bond order and the electronic structure of molecules.
- Ab Initio Calculations
Ab initio calculations start from the fundamental principles of quantum mechanics without relying on experimental data. These calculations provide highly accurate bond orders and other molecular properties, making them valuable for studying complex systems and predicting the properties of new materials.
Quantum chemistry calculations extend our ability to find bond order examples by providing detailed insights into the electronic structure and bonding of molecules. By combining theoretical calculations with experimental techniques, we gain a comprehensive understanding of chemical bonding and its implications in various scientific disciplines.
Experimental Measurements
Experimental measurements play a crucial role in “how to find bond order examples” by providing empirical data that can be used to validate theoretical calculations and models. These measurements involve various techniques that probe the physical and chemical properties of molecules, allowing scientists to determine bond orders experimentally.
One common experimental technique is vibrational spectroscopy, which measures the absorption or emission of infrared or Raman radiation by molecules. By analyzing the vibrational frequencies of different bonds, scientists can infer the bond order and strength. For instance, stronger bonds typically exhibit higher vibrational frequencies. Another technique is electron paramagnetic resonance (EPR) spectroscopy, which is used to study molecules with unpaired electrons. The EPR spectrum provides information about the number and type of unpaired electrons, which can be related to the bond order in the molecule.
Experimental measurements are critical for validating and refining theoretical models of bonding. By comparing experimental data with theoretical predictions, scientists can assess the accuracy of their models and make necessary adjustments. This iterative process leads to a deeper understanding of the nature of chemical bonding and the factors that influence bond order.
In conclusion, experimental measurements provide essential data for “how to find bond order examples.” By combining experimental techniques with theoretical calculations, scientists gain a comprehensive understanding of chemical bonding and its implications in various scientific disciplines, ranging from chemistry and physics to materials science and biology.
Periodic Trends
Periodic trends offer valuable insights into “how to find bond order examples” by establishing relationships between the properties of elements and their position on the periodic table. These trends govern the behavior of elements and their tendency to form bonds, providing a systematic framework for understanding and predicting bond order.
- Electronegativity
Electronegativity measures the ability of an atom to attract electrons. Elements with higher electronegativity tend to form bonds with higher bond orders, as they have a stronger pull on the shared electrons.
- Atomic Radius
Atomic radius influences bond order by determining the distance between atoms. Smaller atoms tend to form bonds with higher bond orders due to their closer proximity and increased overlap of atomic orbitals.
- Ionization Energy
Ionization energy represents the energy required to remove an electron from an atom. Elements with lower ionization energies tend to form bonds with higher bond orders, as they can more easily donate electrons to the bond.
- Valence Electrons
The number of valence electrons dictates the bonding capacity of an element. Elements with more valence electrons can form bonds with higher bond orders, as they have more electrons available for sharing.
Understanding these periodic trends allows us to make informed predictions about bond order based on the properties of the constituent atoms. By considering factors such as electronegativity, atomic radius, ionization energy, and valence electrons, we can gain insights into the nature and strength of chemical bonds, providing valuable guidance for “how to find bond order examples.”
Resonance Structures
Resonance structures play a crucial role in understanding “how to find bond order examples” because they represent the delocalization of electrons within a molecule. By considering resonance structures, we can gain insights into the overall bonding and electron distribution, which directly influences the bond order of the molecule.
When a molecule or ion has multiple Lewis structures that contribute to its overall bonding, we can use resonance structures to describe this delocalization. Resonance structures are different representations of the same molecule, each showing a different arrangement of double and single bonds. The actual structure of the molecule is a hybrid of all the resonance structures, with the bond order being an average of the bond orders in each resonance structure.
For example, in the case of benzene, we can draw two resonance structures that show the alternating single and double bonds around the ring. The true structure of benzene is a resonance hybrid of these two structures, with each carbon-carbon bond having a bond order of 1.5, indicating the delocalization of electrons around the ring.
Understanding resonance structures is essential for finding bond order examples because it provides a more accurate representation of the bonding within a molecule. By considering resonance structures, we can better understand the electron distribution and predict the properties and reactivity of molecules.
Hybridization
Hybridization is a fundamental concept in chemistry that helps explain the bonding and geometry of molecules. It provides a framework for understanding the nature of chemical bonds and their relationship to the properties of molecules. Hybridization is crucial for accurately finding bond order examples, as it allows us to determine the type and strength of bonds formed between atoms.
- Types of Hybridization
There are different types of hybridization, including sp, sp2, sp3, and sp3d. Each type of hybridization results in a specific molecular geometry and bonding pattern.
- Molecular Orbitals
Hybridization involves the mixing of atomic orbitals to form new hybrid orbitals with different shapes and energies. These hybrid orbitals then overlap to form molecular orbitals, which determine the bonding properties of the molecule.
- Bond Order
The bond order of a bond is influenced by the type of hybridization of the atoms involved. For instance, sp3 hybridization typically leads to single bonds, while sp2 hybridization can result in double bonds.
- Examples
Examples of hybridization can be found in various molecules, such as methane (CH4), ethene (C2H4), and benzene (C6H6). By understanding the hybridization of the carbon atoms in these molecules, we can determine their bond orders and explain their molecular properties.
In summary, hybridization plays a critical role in “how to find bond order examples.” By considering the type of hybridization, molecular orbitals formed, and its impact on bond order, we gain a deeper understanding of the electronic structure and bonding behavior of molecules.
FAQs on How to Find Bond Order Examples
The following FAQs address common questions and misconceptions about “how to find bond order examples”:
Question 1: What is bond order and why is it important?
Answer: Bond order is a measure of the strength and type of chemical bond between atoms. It is important for understanding molecular properties such as stability, reactivity, and magnetic behavior.
Question 2: What methods can be used to find bond order examples?
Answer: Bond order examples can be found using various methods, including molecular orbital theory, valence bond theory, X-ray crystallography, and quantum chemistry calculations.
Question 3: How does electronegativity influence bond order?
Answer: Electronegativity measures the ability of an atom to attract electrons. Atoms with higher electronegativity tend to form bonds with higher bond orders.
Question 4: What is the relationship between hybridization and bond order?
Answer: Hybridization involves the mixing of atomic orbitals to form new hybrid orbitals. The type of hybridization influences the bond order of the bonds formed between atoms.
Question 5: How can experimental measurements be used to find bond order examples?
Answer: Experimental measurements, such as vibrational spectroscopy and electron paramagnetic resonance, can provide empirical data that can be used to validate and refine theoretical models of bonding and determine bond orders.
Question 6: What are resonance structures and how do they relate to bond order?
Answer: Resonance structures are different representations of the same molecule, each showing a different arrangement of double and single bonds. The true structure of the molecule is a hybrid of all the resonance structures, with the bond order being an average of the bond orders in each resonance structure.
These FAQs provide a concise overview of key concepts related to “how to find bond order examples.” By understanding these concepts, we can gain insights into the nature of chemical bonding and its implications in various scientific disciplines.
In the next section, we will delve deeper into the theoretical frameworks and practical applications of bond order determination, exploring advanced concepts and their significance in chemistry.
Tips for Finding Bond Order Examples
Understanding how to find bond order examples is crucial for comprehending chemical bonding and molecular properties. Here are some practical tips to guide you:
Tip 1: Utilize molecular orbital theory to visualize and analyze the molecular orbitals involved in bonding.
Tip 2: Apply valence bond theory to determine the hybridization of atoms and predict bond order based on the number and type of atomic orbitals involved.
Tip 3: Employ X-ray crystallography to obtain precise structural data and determine bond lengths, which can provide insights into bond order.
Tip 4: Leverage nuclear magnetic resonance (NMR) spectroscopy to probe the electronic environment of atoms and deduce bond order through chemical shifts and coupling constants.
Tip 5: Utilize quantum chemistry calculations to model molecular systems and calculate bond orders accurately using sophisticated computational methods.
Tip 6: Consider periodic trends to understand how electronegativity, atomic radius, and other properties influence bond order.
Tip 7: Analyze resonance structures to account for electron delocalization and determine the average bond order in resonance hybrids.
Tip 8: Combine experimental measurements with theoretical calculations to validate and refine bond order determinations.
By following these tips, you can effectively find bond order examples and deepen your understanding of chemical bonding. This knowledge is essential for exploring advanced concepts and applications of bond order in various scientific fields.
In the concluding section, we will discuss the significance of bond order in predicting molecular properties, understanding reaction mechanisms, and designing new materials.
Conclusion
This article has provided an in-depth exploration of “how to find bond order examples,” highlighting key theoretical frameworks and practical techniques. By understanding the concepts of molecular orbital theory, valence bond theory, X-ray crystallography, NMR spectroscopy, and quantum chemistry calculations, we can effectively determine bond orders and gain insights into the nature of chemical bonding.
The significance of bond order cannot be overstated. It allows us to predict molecular properties such as stability, reactivity, and magnetic behavior. Moreover, understanding bond order is crucial for comprehending reaction mechanisms and designing new materials with tailored properties. Continued research in this field will further advance our knowledge of chemical bonding and its implications in various scientific disciplines.
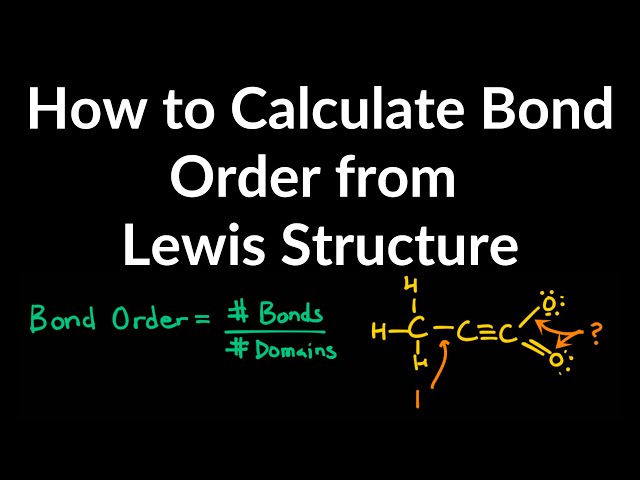