Bond order, a fundamental concept in chemistry, measures the strength of covalent chemical bonds. Understanding how to find bond order is essential for predicting the properties and behavior of molecules.
Bond order influences various molecular characteristics, such as bond length, vibrational frequency, and reactivity. Its determination has been a significant advancement in chemistry, allowing scientists to comprehend and manipulate chemical structures.
This article will delve into the methods for finding bond order in chemistry, exploring its applications and providing practical guidelines for its calculation.
How to Find Bond Order in Chemistry
Determining bond order is crucial for understanding the properties and behavior of molecules. Key aspects to consider include:
- Molecular orbital theory
- Electron configuration
- Resonance structures
- Formal charge
- Hybridization
- Bond length
- Bond strength
- Reactivity
Understanding these aspects enables chemists to predict and explain a wide range of chemical phenomena. For instance, resonance structures help determine the stability of molecules, while hybridization influences the geometry and reactivity of compounds.
Molecular Orbital Theory
Molecular orbital theory (MOT) is a fundamental theory in quantum chemistry that describes the electronic structure of molecules. It provides a framework for understanding how electrons are distributed within molecules and how this distribution determines the chemical bonding and properties of the molecule. MOT is crucial for understanding how to find bond order in chemistry because it provides the theoretical basis for calculating the number of bonding and antibonding electrons in a molecule, which directly determines the bond order.
According to MOT, electrons in a molecule occupy molecular orbitals, which are formed by the linear combination of atomic orbitals. The molecular orbitals can be classified as bonding orbitals, which have lower energy than the atomic orbitals from which they are formed, and antibonding orbitals, which have higher energy. The bond order is determined by the difference between the number of bonding and antibonding electrons. A bond order of 1 indicates a single bond, a bond order of 2 indicates a double bond, and so on.
MOT has wide applications in chemistry. It is used to predict the stability, reactivity, and magnetic properties of molecules. It is also used to design new molecules with desired properties. For example, MOT has been used to design new materials for solar cells, batteries, and catalysts.
Electron configuration
Electron configuration plays a crucial role in determining bond order in chemistry. Bond order, which measures the strength and nature of chemical bonds, is directly related to the number of bonding and antibonding electrons in a molecule. Electron configuration provides the foundation for understanding how electrons are distributed within molecules, enabling chemists to determine the number of bonding and antibonding electrons and, subsequently, the bond order.
For instance, consider the formation of a bond between two hydrogen atoms. Each hydrogen atom has one electron, and when these atoms come together to form a molecule, their atomic orbitals overlap, resulting in the formation of molecular orbitals. The electron configuration of the hydrogen molecule is 1s2, where the superscript 2 indicates that the 1s molecular orbital is filled with two electrons. Since both electrons are in bonding orbitals, the bond order of the hydrogen molecule is 1, indicating a single bond.
The relationship between electron configuration and bond order has wide-ranging applications in chemistry. By manipulating the electron configuration of atoms, chemists can control the bond order and, consequently, the properties of molecules. This understanding is essential for designing new materials with tailored properties, such as strength, reactivity, and conductivity.
Resonance structures
Resonance structures, a fundamental concept in chemistry, provide a means to represent the delocalization of electrons within certain molecules or polyatomic ions. These structures are crucial for understanding how to find bond order in chemistry, as they offer insights into the distribution of electrons and the nature of chemical bonds.
Resonance structures arise when a molecule or ion has multiple Lewis structures, each of which represents a valid arrangement of electrons. The resonance structures differ in the placement of double and single bonds, indicating the resonance hybrid, which is a more accurate representation of the molecule’s electronic structure. In such cases, the bond order is not an integer but rather a weighted average of the bond orders in the resonance structures.
For example, the carbonate ion (CO32-) has three resonance structures, each depicting a different arrangement of double bonds between the carbon atom and the three oxygen atoms. The resonance hybrid shows that the carbon-oxygen bond order is 1.33, indicating a delocalized electronic structure with partial double bond character between the carbon and each oxygen atom.
Understanding resonance structures is essential for accurately determining bond orders and predicting the properties and reactivity of molecules. It finds applications in various fields, including organic chemistry, inorganic chemistry, and biochemistry. By considering resonance structures, chemists can gain insights into the stability, reactivity, and spectroscopic properties of chemical species.
Formal Charge
Formal charge, a crucial concept in chemistry, plays a significant role in determining bond order and understanding the electronic structure of molecules. It assigns a hypothetical charge to each atom in a molecule, providing insights into the distribution of electrons and the polarity of bonds.
- Definition: Formal charge is the hypothetical charge assigned to an atom in a molecule, calculated as the difference between the number of valence electrons in the free atom and the number of electrons assigned to that atom in the Lewis structure.
- Significance: Formal charge helps determine the stability and reactivity of molecules. Molecules with formal charges on atoms tend to be less stable and more reactive.
- Applications: Formal charge is used to determine the most likely Lewis structure for a molecule, predict the polarity of bonds, and estimate the stability of resonance structures.
- Limitations: Formal charge is a theoretical concept that does not always accurately reflect the actual charge distribution in a molecule. It should be used in conjunction with other methods to obtain a comprehensive understanding of molecular electronic structure.
Understanding formal charge is essential for accurately determining bond orders and predicting the properties and reactivity of molecules. It provides valuable insights into the electronic structure and bonding characteristics of chemical species.
Hybridization
Hybridization, a cornerstone of valence bond theory, plays a crucial role in determining bond order in chemistry. It involves the intermixing of atomic orbitals to form new hybrid orbitals with distinct shapes and energies, influencing the geometry and properties of molecules.
- Types of Hybridization
Hybridization occurs in various forms, with the most common being sp, sp2, sp3, and sp3d hybridization. Each type involves the combination of a specific number and type of atomic orbitals, resulting in hybrid orbitals with unique geometries.
- Orbital Overlap
The extent of orbital overlap between hybrid orbitals determines the strength and type of chemical bonds formed. Overlapping hybrid orbitals form sigma () and pi () bonds, influencing the bond order and molecular shape.
- Bond Angles and Molecular Geometry
The geometry of a molecule is dictated by the hybridization of its constituent atoms. Different types of hybridization result in specific bond angles and molecular shapes, such as linear, trigonal planar, or tetrahedral.
- Bond Order and Properties
Hybridization directly affects bond order, which in turn influences the properties of molecules. For instance, compounds with sp3 hybridized carbon atoms tend to have higher bond orders and greater stability compared to those with sp2 or sp hybridized carbon atoms.
Understanding hybridization is essential for accurately determining bond orders and predicting the structure, properties, and reactivity of molecules. It provides a deeper insight into the electronic structure and bonding characteristics of chemical species.
Bond Length
Bond length, a fundamental property of chemical bonds, plays a crucial role in determining bond order in chemistry. The relationship between bond length and bond order is inverse; as bond order increases, bond length decreases. This relationship arises from the quantum mechanical properties of molecular orbitals, which are the regions around atoms where electrons are most likely to be found.
In covalent bonds, electrons are shared between atoms, forming molecular orbitals. The strength of a covalent bond is determined by the overlap of these molecular orbitals. A greater overlap results in a stronger bond and a shorter bond length. Bond order, on the other hand, is a measure of the number of electron pairs shared between two atoms. A higher bond order indicates more electron pairs shared, leading to a stronger bond and a shorter bond length.
Understanding the relationship between bond length and bond order is crucial for predicting the properties and reactivity of molecules. For example, in carbon-carbon bonds, a triple bond has a shorter bond length and higher bond order compared to a double bond, which in turn has a shorter bond length and higher bond order than a single bond. This understanding allows chemists to tailor the properties of molecules by manipulating bond lengths and bond orders.
Bond Strength
Bond strength, a fundamental property of chemical bonds, plays a critical role in determining the stability and reactivity of molecules. Understanding bond strength is essential for accurately finding bond order in chemistry and predicting the properties of chemical compounds.
Bond strength is directly related to bond order. A higher bond order indicates a stronger bond, and a stronger bond has a shorter bond length. The strength of a bond is determined by the number of electrons shared between the bonded atoms and the overlap of their atomic orbitals. A greater overlap of atomic orbitals leads to a stronger bond. Bond strength can be measured experimentally using various techniques such as infrared spectroscopy, nuclear magnetic resonance spectroscopy, and mass spectrometry.
In the context of finding bond order in chemistry, bond strength is a critical component. By understanding the relationship between bond strength and bond order, chemists can accurately predict the bond order of a molecule based on its bond strength. This understanding is crucial for predicting the properties and reactivity of molecules and designing new materials with desired properties.
Reactivity
Reactivity, a defining characteristic of chemical species, plays a crucial role in determining the course of chemical reactions and the properties of molecules. Understanding reactivity is essential for finding bond order in chemistry, as it provides insights into the strength and behavior of chemical bonds.
Reactivity is directly influenced by bond order. A higher bond order indicates a stronger bond, which in turn leads to lower reactivity. This is because stronger bonds require more energy to break, making the molecule less reactive. For example, alkenes (compounds with carbon-carbon double bonds) are less reactive than alkanes (compounds with carbon-carbon single bonds) due to the higher bond order in the double bond.
Finding bond order in chemistry is critical for predicting reactivity and understanding the behavior of molecules. By determining bond order, chemists can gain insights into the stability, reactivity, and reaction pathways of chemical compounds. This understanding has practical applications in various fields, including organic chemistry, inorganic chemistry, and biochemistry.
Frequently Asked Questions
This section addresses common questions and clarifies aspects of finding bond order in chemistry.
Question 1: What is bond order, and why is it important?
Answer: Bond order describes the strength of a chemical bond and predicts the properties and reactivity of molecules. It indicates the number of electron pairs shared between bonded atoms.
Question 2: How do I determine the bond order of a molecule?
Answer: To find bond order, count the number of bonding electron pairs and subtract the number of antibonding electron pairs. The result is the bond order.
Question 3: What factors influence bond order?
Answer: Factors affecting bond order include the number of shared electrons, the electronegativity of the bonded atoms, and resonance.
Question 4: How is bond order related to bond length and strength?
Answer: Bond order is inversely related to bond length and directly related to bond strength. Higher bond order means shorter and stronger bonds.
Question 5: Can bond order be used to predict the reactivity of a molecule?
Answer: Yes, bond order can indicate the reactivity of a molecule. Higher bond order generally corresponds to lower reactivity.
Question 6: How do I find the bond order of a molecule with resonance structures?
Answer: For resonance structures, calculate the bond order for each resonance structure and take the average to find the overall bond order.
These FAQs provide essential insights into finding bond order in chemistry. Understanding bond order is crucial for comprehending molecular structure, bonding, and reactivity.
In the next section, we will delve deeper into advanced concepts related to bond order, exploring its applications in various fields of chemistry.
Tips for Finding Bond Order in Chemistry
This section provides practical tips to help you accurately find bond order in chemistry, enabling you to better understand and predict molecular behavior.
Tip 1: Determine the molecular orbital diagram and identify the number of bonding and antibonding electrons.
Tip 2: Use electron configuration to count the number of valence electrons and distribute them into molecular orbitals.
Tip 3: Consider resonance structures to account for delocalization of electrons and calculate the average bond order.
Tip 4: Determine the formal charge of each atom to assess electron distribution and identify potential resonance structures.
Tip 5: Understand the hybridization of atoms, as it influences the geometry and bond order of molecules.
Tip 6: Measure bond length experimentally, as a shorter bond length typically indicates a higher bond order.
Tip 7: Analyze bond strength, as stronger bonds generally correspond to higher bond orders.
Tip 8: Relate bond order to reactivity, as higher bond order often implies lower reactivity.
By following these tips, you can effectively find bond order in chemistry, gaining valuable insights into molecular structure and properties. This understanding is crucial for predicting reactivity, designing new materials, and comprehending various chemical phenomena.
In the next section, we will explore advanced applications of bond order in different fields of chemistry, demonstrating its significance in understanding complex chemical systems.
Conclusion
In this article, we have explored the topic of “how to find bond order in chemistry,” examining various methods and concepts. We highlighted the importance of understanding molecular orbital theory, electron configuration, resonance structures, formal charge, hybridization, bond length, bond strength, and reactivity in determining bond order.
Key points to remember include the inverse relationship between bond order and bond length, the direct relationship between bond order and bond strength, and the influence of bond order on molecular reactivity. These concepts are interconnected and provide a comprehensive understanding of chemical bonding.
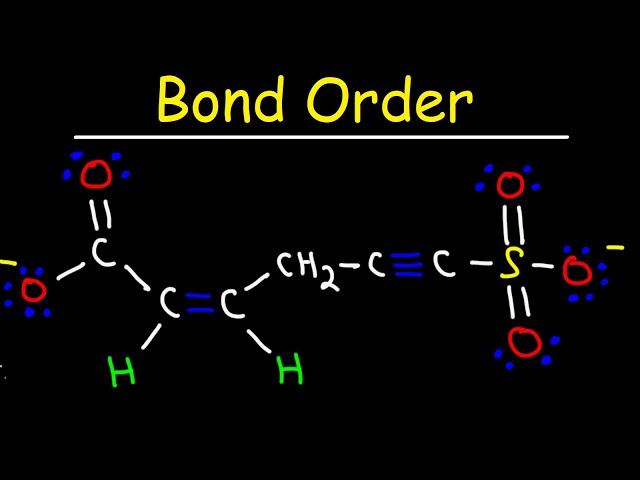