Discovering bond order in resonance structures is a technique for comprehending the electronic structure of molecules.
This concept is vital in chemistry as it elucidates the behavior and properties of various chemical compounds. Historically, the development of resonance theory by Linus Pauling revolutionized our understanding of chemical bonding.
This article delves into the intricacies of determining bond order in resonance structures, exploring theoretical principles and practical applications.
How to Find Bond Order in Resonance Structures
Determining bond order in resonance structures is essential for understanding the electronic structure and properties of molecules.
- Identify Resonance Structures
- Calculate Formal Charges
- Consider Lewis Structures
- Apply the Kekule Structure
- Examine Bond Lengths
- Analyze Bond Angles
- Use Spectroscopic Data
- Employ Computational Chemistry
- Consider Molecular Orbital Theory
- Apply Valence Bond Theory
These aspects provide a comprehensive framework for determining bond order in resonance structures, enabling chemists to gain insights into the nature of chemical bonding and molecular behavior.
Identify Resonance Structures
Identifying resonance structures is a foundational step in determining bond order in resonance structures, a technique that provides valuable insights into the electronic structure and properties of molecules.
- Drawing Lewis Structures: Resonance structures are based on Lewis structures, which depict the arrangement of atoms and bonds within a molecule.
- Identifying Resonance Contributors: Resonance structures are sets of Lewis structures that represent the different possible electron arrangements for a given molecule.
- Counting Resonance Structures: The number of resonance structures for a molecule can vary, and this number can provide insights into the stability and reactivity of the molecule.
- Evaluating Resonance Structures: Not all resonance structures are equally important, and resonance theory provides methods for evaluating the relative contributions of different resonance structures to the overall electronic structure of a molecule.
By identifying resonance structures, chemists can gain a deeper understanding of the electronic structure and properties of molecules, including bond order, molecular stability, and reactivity.
Calculate Formal Charges
Calculating formal charges is a crucial step in determining bond order in resonance structures. Formal charges are assigned to each atom in a molecule to represent the hypothetical charge that atom would have if all bonds in the molecule were purely ionic. These charges provide insights into the distribution of electrons within the molecule and can help identify the most likely resonance structures.
To calculate formal charges, the following formula is used: Formal Charge = (Number of Valence Electrons) – (Number of Non-bonding Electrons) – (1/2 * Number of Bonding Electrons)
By calculating formal charges for each atom in a molecule, chemists can identify atoms with significant positive or negative charges. These charged atoms are more likely to participate in resonance, as they can stabilize the molecule by distributing the charges more evenly. Resonance structures with the lowest formal charges are generally more stable and contribute more to the overall electronic structure of the molecule.
In summary, calculating formal charges is a fundamental step in determining bond order in resonance structures. By assigning formal charges to each atom, chemists can identify the most likely resonance structures and gain insights into the distribution of electrons within the molecule.
Consider Lewis Structures
Considering Lewis structures is a fundamental step in determining bond order in resonance structures. Lewis structures provide a representation of the bonding and arrangement of atoms in a molecule, enabling chemists to visualize and analyze the distribution of electrons.
- Identify Atoms and Bonds: Lewis structures depict the connectivity of atoms and the types of bonds between them, providing a starting point for understanding the electronic structure of the molecule.
- Count Valence Electrons: The number of valence electrons in a molecule determines its bonding capacity and can be used to identify potential resonance structures.
- Determine Formal Charges: Formal charges assigned to atoms in Lewis structures provide insights into the distribution of electrons and can help identify the most stable resonance structures.
- Analyze Resonance: Lewis structures allow for the visualization of resonance structures, where electrons are delocalized over multiple atoms, providing a deeper understanding of the electronic structure and properties of the molecule.
By considering Lewis structures, chemists can gain valuable insights into the electronic structure and bonding of molecules, which are essential for determining bond order in resonance structures. These insights enable chemists to predict the properties and reactivity of molecules, as well as design new molecules with desired characteristics.
Apply the Kekule Structure
Applying the Kekule structure is a fundamental step in determining bond order in resonance structures. It involves drawing a Lewis structure that alternates single and double bonds between the atoms in a molecule, providing a framework for understanding the resonance and delocalization of electrons.
- Identifying Resonance Contributors: The Kekule structure provides a starting point for identifying resonance contributors, which are different Lewis structures that represent the resonance hybrid of the molecule.
- Calculating Bond Order: By analyzing the Kekule structures, chemists can determine the bond order in resonance structures. Bond order is an indication of the strength and nature of the bond between two atoms.
- Understanding Delocalization: The Kekule structure helps visualize the delocalization of electrons in resonance structures. Delocalization occurs when electrons are spread over multiple atoms, resulting in fractional bond orders.
- Predicting Molecular Properties: Applying the Kekule structure allows chemists to predict the molecular properties and reactivity of compounds. For example, the bond order can provide insights into the stability, polarity, and spectroscopic properties of the molecule.
In summary, applying the Kekule structure is a powerful tool for determining bond order in resonance structures. It provides a visual representation of the resonance and delocalization of electrons, enabling chemists to make predictions about the properties and behavior of molecules.
Examine Bond Lengths
Examining bond lengths is a critical step in determining bond order in resonance structures. Bond length refers to the distance between the nuclei of two bonded atoms, and it is inversely proportional to the bond order. Therefore, by measuring or estimating bond lengths, chemists can gain insights into the bond order in resonance structures.
In resonance structures, the delocalization of electrons leads to fractional bond orders. As a result, the bond lengths in resonance structures are typically intermediate between single and double bonds. For example, in the resonance structures of benzene, the C-C bond lengths are approximately 1.39 , which is between the typical C-C single bond length (1.54 ) and the typical C-C double bond length (1.34 ).
Examining bond lengths is particularly useful in confirming the resonance hybrid and determining the relative contributions of different resonance structures. Resonance structures with shorter bond lengths are generally more important contributors to the overall electronic structure of the molecule. By analyzing bond lengths, chemists can gain a deeper understanding of the electronic structure and properties of molecules, including their stability, reactivity, and spectroscopic properties.
Analyze Bond Angles
Analyzing bond angles is another valuable step in determining bond order in resonance structures. Bond angles provide insights into the geometry of the molecule and the hybridization of the atoms involved in the resonance. Resonance structures often involve multiple atoms with trigonal planar or tetrahedral geometry, and the bond angles can help identify the most stable resonance contributor.
In resonance structures, the delocalization of electrons can lead to changes in bond angles. For example, in the resonance structures of benzene, the C-C-C bond angles are approximately 120, which is consistent with the trigonal planar geometry of the carbon atoms. This observation supports the resonance hybrid model and indicates that the electrons are delocalized over the entire ring.
Analyzing bond angles can be particularly useful in cases where there are competing resonance structures with different geometries. By examining the bond angles, chemists can determine which resonance structure is more likely to be the major contributor to the overall electronic structure of the molecule. This understanding can help predict the molecular properties, reactivity, and spectroscopic behavior of the compound.
Use Spectroscopic Data
Spectroscopic data provide valuable insights into the electronic structure of molecules, including bond order in resonance structures. Spectroscopic techniques measure the interaction of electromagnetic radiation with molecules, allowing for the identification and characterization of different molecular properties.
- UV-Visible Spectroscopy: UV-visible spectroscopy measures the absorption of ultraviolet and visible light by molecules. The absorption bands in the spectrum correspond to electronic transitions, which can provide information about the energy levels and bonding within the molecule.
- Infrared Spectroscopy: Infrared spectroscopy measures the absorption of infrared radiation by molecules. The absorption bands in the spectrum correspond to vibrational modes, which can provide information about the bond strengths and functional groups present in the molecule.
- NMR Spectroscopy: NMR spectroscopy measures the magnetic properties of atomic nuclei. The chemical shifts in the NMR spectrum correspond to the electron density around the nuclei, which can provide information about the hybridization and bonding of the atoms.
- Mass Spectrometry: Mass spectrometry measures the mass-to-charge ratio of ions. The fragmentation patterns in the mass spectrum can provide information about the molecular structure and bonding, including the identification of resonance structures.
By combining spectroscopic data from different techniques, chemists can gain a comprehensive understanding of the electronic structure and bonding in resonance structures. This information can be used to determine bond order, predict molecular properties, and design new molecules with specific properties.
Employ Computational Chemistry
Computational chemistry plays a pivotal role in determining bond order in resonance structures. It provides powerful computational tools and methods for simulating and analyzing molecular systems, enabling chemists to gain insights into the electronic structure and bonding of molecules.
Computational chemistry allows chemists to calculate the wavefunction of a molecule, which describes the distribution of electrons within the molecule. From the wavefunction, various properties can be computed, including bond orders. By employing sophisticated algorithms and high-performance computing resources, computational chemistry can handle complex molecular systems and provide accurate estimates of bond orders in resonance structures.
One of the prominent applications of computational chemistry in this context is density functional theory (DFT). DFT is a widely used method for calculating the electronic structure of molecules, and it has been successfully applied to determine bond orders in resonance structures. DFT calculations can provide detailed information about the electron density distribution and the nature of chemical bonds, allowing chemists to understand the stability and reactivity of resonance structures.
Overall, computational chemistry is a valuable tool for determining bond order in resonance structures. It provides accurate and reliable estimates of bond orders, enabling chemists to gain a deeper understanding of the electronic structure and bonding of molecules. This understanding is essential for predicting molecular properties, designing new materials, and developing new drugs and therapies.
Consider Molecular Orbital Theory
Molecular orbital theory (MOT) provides a theoretical framework for understanding the electronic structure of molecules, including resonance structures. MOT describes the molecular orbitals as mathematical functions that represent the wave-like behavior of electrons within the molecule. These molecular orbitals are formed by the linear combination of atomic orbitals, and their shapes and energies determine the chemical bonding and properties of the molecule.
In the context of resonance structures, MOT plays a crucial role in determining bond order. Bond order is a measure of the strength and multiplicity of the bond between two atoms, and it can be calculated based on the molecular orbitals involved in the bond. By analyzing the molecular orbitals and their interactions, chemists can gain insights into the delocalization of electrons and the resonance stabilization of the molecule.
Real-life examples of MOT applications in determining bond order in resonance structures include the study of benzene and other aromatic compounds. Benzene, with its six-membered ring structure, exhibits resonance between multiple Kekule structures. MOT analysis reveals that the molecular orbitals of benzene are delocalized over the entire ring, resulting in equal bond lengths between all carbon atoms. This delocalization contributes to the stability and unique properties of benzene.
Understanding the connection between MOT and bond order in resonance structures has practical applications in various fields, such as materials science, drug design, and computational chemistry. By accurately determining bond order, chemists can predict molecular properties, design new materials with specific electronic and structural characteristics, and optimize drug molecules for better efficacy and reduced side effects.
Apply Valence Bond Theory
Valence bond theory (VBT) is a powerful tool for understanding the electronic structure and bonding in molecules, including resonance structures. VBT describes chemical bonding as the overlap of atomic orbitals, leading to the formation of molecular orbitals. These molecular orbitals can be used to determine the bond order in resonance structures, providing insights into the strength and nature of the chemical bonds.
One key aspect of VBT is the concept of hybridization, which explains how atomic orbitals combine to form new hybrid orbitals with specific shapes and energies. In resonance structures, VBT can be used to determine the hybridization of the atoms involved in the resonance and how this hybridization affects the bond order. For example, in the benzene molecule, VBT shows that the carbon atoms are sp2 hybridized, leading to the formation of three bonds and one bond between each pair of carbon atoms. This hybridization contributes to the resonance stabilization of the benzene ring.
VBT also provides a framework for understanding the electronic configuration of molecules and the stability of different resonance structures. By analyzing the molecular orbitals and their interactions, chemists can determine the relative contributions of different resonance structures to the overall electronic structure of the molecule. This understanding is crucial for predicting molecular properties, such as bond lengths, bond angles, and reactivity.
In summary, applying valence bond theory is a critical component of how to find bond order in resonance structures. VBT provides a theoretical framework for understanding the electronic structure and bonding in molecules, allowing chemists to determine the hybridization of atoms, analyze molecular orbitals, and predict the stability of resonance structures. This understanding has practical applications in various fields, including organic chemistry, inorganic chemistry, and materials science.
Frequently Asked Questions about Bond Order in Resonance Structures
This section addresses common questions and clarifies aspects related to determining bond order in resonance structures.
Question 1: What is bond order, and why is it important?
Answer: Bond order refers to the strength and multiplicity of the bond between two atoms. It is crucial for understanding the electronic structure and properties of molecules, such as stability, reactivity, and molecular geometry.
Question 2: How do I identify resonance structures for a molecule?
Answer: Resonance structures are different Lewis structures that represent the delocalization of electrons within a molecule. To identify resonance structures, start by drawing the Lewis structure and look for alternative arrangements of double bonds and lone pairs that maintain the same number of electrons and atoms.
Question 3: What is the relationship between resonance and bond order?
Answer: Resonance delocalization leads to fractional bond orders, indicating that the bond strength falls between single and double bonds. Resonance structures with lower bond orders contribute more significantly to the overall electronic structure of the molecule.
Question 4: How can I calculate bond order in resonance structures?
Answer: Bond order can be calculated using various methods, including the Kekule structure, examination of bond lengths and angles, spectroscopic data, computational chemistry, molecular orbital theory, and valence bond theory.
Question 5: What are the limitations of determining bond order in resonance structures?
Answer: While these methods provide valuable insights, it’s important to note that resonance is a theoretical concept, and the exact bond order may vary depending on the chosen method and the specific molecule under consideration.
Question 6: How does understanding bond order help in predicting molecular properties?
Answer: Determining bond order allows chemists to predict molecular properties such as stability, polarity, reactivity, and spectroscopic behavior. This understanding is essential for designing new materials, optimizing drug molecules, and comprehending chemical reactions.
These FAQs provide a concise overview of key concepts and methods related to bond order in resonance structures, setting the stage for further exploration and application in various chemical contexts.
In the next section, we will delve into advanced techniques and applications of determining bond order in resonance structures, exploring how this knowledge contributes to our understanding of molecular behavior and properties.
Tips for Determining Bond Order in Resonance Structures
This section provides practical tips to assist you in effectively determining bond order in resonance structures. By following these guidelines, you can refine your understanding and enhance your ability to analyze and predict molecular properties.
Tip 1: Identify Resonance Structures Systematically
Start by drawing the Lewis structure and explore alternative arrangements of double bonds and lone pairs that maintain the same total number of electrons and atoms.
Tip 2: Calculate Formal Charges
Assign formal charges to each atom in the resonance structures to assess their relative stability. Structures with lower formal charges contribute more significantly to the overall electronic structure.
Tip 3: Utilize Spectroscopic Data
Analyze experimental data from UV-visible, infrared, and NMR spectroscopy to gather insights into the electronic transitions and molecular vibrations, which can provide evidence for resonance.
Tip 4: Examine Bond Lengths and Angles
Compare bond lengths and angles to typical values for single and double bonds. Resonance structures often exhibit intermediate values, indicating delocalization of electrons.
Tip 5: Apply Molecular Orbital Theory
Use molecular orbital theory to calculate electron density distribution and understand the interactions between orbitals. This approach provides a deeper understanding of resonance and bond order.
Tip 6: Leverage Computational Chemistry
Employ computational methods such as density functional theory (DFT) to obtain accurate estimates of bond orders and visualize molecular orbitals. These tools can handle complex molecular systems.
Tip 7: Consider Valence Bond Theory
Analyze the hybridization of atoms and the overlap of atomic orbitals using valence bond theory. This approach helps determine the strength and nature of bonds in resonance structures.
These tips empower you to navigate the complexities of resonance structures, enabling you to determine bond order effectively. They provide a solid foundation for further exploration and application in understanding molecular behavior and properties.
In the concluding section, we will explore advanced techniques and applications of determining bond order in resonance structures, highlighting their significance in various chemical contexts.
Conclusion
In summary, determining bond order in resonance structures is a multifaceted process that provides valuable insights into the electronic structure and properties of molecules. Key ideas explored in this article include the identification of resonance structures, calculation of formal charges, and utilization of spectroscopic data, bond lengths and angles, molecular orbital theory, computational chemistry, and valence bond theory.
These techniques are interconnected and complementary, allowing chemists to accurately determine bond order and understand the delocalization of electrons in resonance structures. This knowledge is crucial for predicting molecular properties, designing new materials, and comprehending chemical reactions.
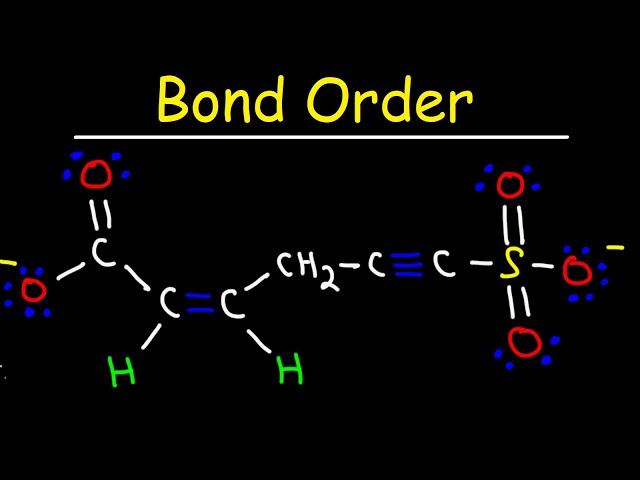