Determining bond order is a crucial aspect of chemistry, as it provides insights into the strength and nature of chemical bonds. One specific instance where bond order determination is vital is for the B2 molecule.
Understanding the bond order of B2 enables chemists to predict its reactivity, stability, and electronic structure. Historically, the concept of bond order was first introduced by Linus Pauling in the 1930s, providing a theoretical framework for comprehending chemical bonding.
In this article, we will delve into the intricacies of determining the bond order of B2, exploring the methods, applications, and implications of this fundamental chemical concept.
How to Find Bond Order of B2
Determining the bond order of B2 is crucial for understanding its chemical properties and behavior. Key aspects to consider include:
- Molecular Orbital Theory
- Valence Bond Theory
- Resonance
- Hybridization
- Bond Length
- Bond Energy
- Magnetic Properties
- Spectroscopic Data
- Computational Chemistry
These aspects provide a comprehensive framework for analyzing and comprehending the bond order of B2. Understanding these concepts enables chemists to predict the stability, reactivity, and electronic structure of B2, contributing to advancements in various fields of chemistry.
Molecular Orbital Theory
Molecular Orbital Theory plays a pivotal role in comprehending the electronic structure of molecules, providing a theoretical foundation for determining bond order.
- Atomic Orbitals
Atomic orbitals, the wave functions describing the behavior of electrons around individual atoms, serve as the building blocks for molecular orbitals. - Linear Combination of Atomic Orbitals (LCAO)
Molecular orbitals arise from the mathematical combination of atomic orbitals, resulting in new orbitals that extend over the entire molecule. - Bonding and Antibonding Orbitals
Molecular orbitals can be classified as bonding or antibonding based on their effect on the electron density between atoms. Bonding orbitals concentrate electron density, strengthening the bond, while antibonding orbitals reduce electron density, weakening the bond. - Molecular Orbital Energy Levels
The energy levels of molecular orbitals determine the stability and reactivity of the molecule. Lower energy orbitals are more stable and less reactive.
By analyzing the molecular orbitals of B2, chemists can determine the bond order, which provides insights into the molecule’s stability, reactivity, and electronic structure. Molecular Orbital Theory serves as a powerful tool for understanding and predicting the behavior of molecules, including B2.
Valence Bond Theory
Valence Bond Theory provides a framework for understanding chemical bonding by considering the interactions between atomic orbitals. In the context of determining the bond order of B2, Valence Bond Theory plays a critical role as it enables the visualization and description of the bonding between the two boron atoms.
The theory proposes that the B-B bond in B2 is formed by the overlap of two sp3 hybrid orbitals, each containing one unpaired electron. This overlap results in the formation of a sigma () bond, which is the strongest type of covalent bond. The strength of the bond is directly related to the amount of overlap between the atomic orbitals, which in turn determines the bond order.
By analyzing the hybridization of the atomic orbitals involved in the B-B bond and the extent of their overlap, Valence Bond Theory provides a qualitative understanding of the bond order in B2. This understanding is essential for predicting the stability, reactivity, and overall properties of the B2 molecule.
Resonance
Resonance is a crucial concept in chemistry, providing a deeper understanding of the electronic structure and bonding within molecules. In the context of determining the bond order of B2, resonance plays a vital role, offering insights into the molecule’s stability and properties.
- Delocalization of Electrons
Resonance involves the delocalization of electrons across multiple atoms or bonds within a molecule. In B2, the electrons are delocalized, resulting in a more stable and symmetrical molecule. - Equivalent Resonance Structures
Resonance structures are different representations of the same molecule, which differ only in the placement of electrons. In B2, there are two equivalent resonance structures, each contributing to the overall electronic structure. - Resonance Hybrid
The actual electronic structure of B2 is a resonance hybrid, a combination of all the contributing resonance structures. This hybrid structure has an average bond order that is intermediate between the single and double bond orders. - Stability and Reactivity
Resonance contributes to the stability of B2 by lowering its overall energy. The delocalization of electrons makes the molecule less reactive, as the electrons are less localized and less likely to participate in reactions.
By considering resonance, chemists gain a more comprehensive view of the electronic structure and bonding in B2. This understanding is essential for accurately determining the bond order and predicting the molecule’s properties and reactivity.
Hybridization
Hybridization plays a pivotal role in determining the bond order of B2, providing a framework for understanding the electronic structure and bonding within the molecule.
- Atomic Orbitals
Hybridization involves the combination of atomic orbitals to form new hybrid orbitals with different shapes and energies. In the case of B2, the two boron atoms undergo sp3 hybridization, resulting in four equivalent hybrid orbitals. - Bond Formation
The sp3 hybrid orbitals on each boron atom overlap to form a bond, the strongest type of covalent bond. The extent of orbital overlap determines the strength and length of the bond, which in turn affects the bond order. - Molecular Geometry
Hybridization influences the molecular geometry of B2. The sp3 hybridization results in a tetrahedral arrangement of the hybrid orbitals, leading to a linear molecular geometry for B2. - Resonance
Hybridization is closely related to resonance in B2. The sp3 hybrid orbitals allow for the delocalization of electrons, contributing to the resonance structures and overall stability of the molecule.
By considering hybridization, chemists gain a deeper understanding of the electronic structure and bonding in B2, enabling them to accurately determine the bond order and predict the molecule’s properties and reactivity.
Bond Length
Bond length is a critical component of determining the bond order of B2. It refers to the distance between the nuclei of the two bonded atoms. A shorter bond length typically indicates a stronger bond and a higher bond order. Conversely, a longer bond length suggests a weaker bond and a lower bond order.
In the case of B2, the bond length is a consequence of the hybridization of the atomic orbitals involved in bonding. The sp3 hybridization of the boron atoms results in a tetrahedral arrangement of the hybrid orbitals, leading to a linear molecular geometry and a bond length of 1.74 . This bond length is consistent with the bond order of 1, indicating a single bond between the two boron atoms.
Understanding the relationship between bond length and bond order is essential for predicting the properties and reactivity of B2. For instance, the short bond length and high bond order in B2 contribute to its high stability and low reactivity. This understanding finds practical applications in various fields, including materials science and nanotechnology, where controlling bond lengths and bond orders is crucial for designing and synthesizing materials with specific properties.
Bond Energy
Bond energy plays a crucial role in determining the bond order of B2, providing insights into the strength and stability of the chemical bond between the two boron atoms.
- Bond Dissociation Energy
Bond dissociation energy is the energy required to break a bond between two atoms in a molecule. In the case of B2, the bond dissociation energy is a measure of the strength of the B-B bond. - Bond Length
Bond energy and bond length are inversely related. A shorter bond length typically corresponds to a stronger bond and higher bond energy. The bond length of B2 provides information about the strength of the B-B bond. - Resonance
Resonance can affect bond energy by delocalizing electrons and lowering the overall energy of the molecule. In B2, resonance contributes to the stability of the molecule and influences the bond energy. - Molecular Orbital Theory
Molecular Orbital Theory provides a theoretical framework for understanding bond energy. By analyzing the molecular orbitals of B2, chemists can gain insights into the electronic structure and bond energy of the molecule.
Understanding bond energy is essential for predicting the reactivity and stability of B2. The bond energy, along with other factors such as bond length and resonance, provides valuable information for determining the bond order of B2 and comprehending its chemical properties.
Magnetic Properties
Magnetic properties play a significant role in understanding the bond order of B2, offering valuable insights into the molecule’s electronic structure and behavior. These properties provide crucial information, complementing other techniques used to determine bond order.
- Paramagnetism
Paramagnetism arises when a molecule possesses unpaired electrons, resulting in a magnetic field. B2 is diamagnetic, indicating the absence of unpaired electrons and, consequently, a bond order of 1. - Nuclear Magnetic Resonance (NMR) Spectroscopy
NMR spectroscopy exploits the magnetic properties of atomic nuclei to provide information about the electronic environment and bond order. By analyzing the NMR spectra of B2, chemists can gain insights into the bonding and molecular structure. - Electron Paramagnetic Resonance (EPR) Spectroscopy
EPR spectroscopy is a powerful tool for studying paramagnetic molecules. B2, being diamagnetic, does not exhibit EPR signals, further supporting the bond order of 1. - Magnetic Susceptibility
Magnetic susceptibility measures the degree to which a material responds to an applied magnetic field. The diamagnetic nature of B2 results in a negative magnetic susceptibility value, consistent with its lack of unpaired electrons and a bond order of 1.
These magnetic properties, when combined with other characterization techniques, provide a comprehensive understanding of the bond order of B2, enabling chemists to probe the molecule’s electronic structure, reactivity, and overall behavior.
Spectroscopic Data
Spectroscopic data plays a crucial role in the determination of bond order in B2. By analyzing the interaction of electromagnetic radiation with the molecule, we can gain insights into its electronic structure and bonding characteristics.
- UV-Visible Spectroscopy
This technique measures the absorption of ultraviolet and visible light by B2, providing information about the electronic transitions and the energy difference between molecular orbitals. - Infrared Spectroscopy
This technique measures the absorption of infrared radiation by B2, providing information about the vibrational modes of the molecule and the strength of the B-B bond. - Raman Spectroscopy
This technique measures the inelastic scattering of light by B2, providing information about the molecular vibrations and the symmetry of the molecule. - Nuclear Magnetic Resonance (NMR) Spectroscopy
This technique measures the magnetic properties of atomic nuclei in B2, providing information about the electronic environment and the bond order.
By combining these spectroscopic techniques, chemists can obtain a comprehensive understanding of the electronic structure and bonding in B2, which is essential for accurately determining its bond order. Spectroscopic data provides valuable insights into the chemical properties and behavior of B2, enabling researchers to tailor its applications in various fields, such as materials science and catalysis.
Computational Chemistry
Computational chemistry plays a significant role in determining the bond order of B2. It involves using computational methods to solve the Schrdinger equation, which describes the wave function and energy of electrons in a molecule. By solving this equation, computational chemistry provides valuable insights into the electronic structure and bonding characteristics of B2.
One of the key applications of computational chemistry in determining the bond order of B2 is through quantum chemical calculations. These calculations use sophisticated algorithms to approximate the wave function of B2 and calculate its total energy. By comparing the energy of different molecular orbitals, chemists can determine the bond order, which is related to the number of electrons occupying bonding orbitals.
Computational chemistry is a powerful tool that enables researchers to study the electronic structure of molecules at a detailed level. By providing accurate and reliable data on bond orders, computational chemistry helps us understand the chemical properties and behavior of B2. This understanding has practical applications in various fields, such as materials science and catalysis, where controlling bond orders is crucial for designing and synthesizing materials with specific properties.
Frequently Asked Questions
This section addresses common questions and uncertainties regarding the determination of bond order in B2.
Question 1: What is the significance of bond order in understanding B2?
Bond order is a fundamental property that provides insights into the strength and nature of the chemical bond between the two boron atoms in B2. It influences the stability, reactivity, and electronic structure of the molecule.
Question 2: What experimental techniques are used to determine bond order?
Various experimental techniques, such as X-ray crystallography, electron diffraction, and spectroscopy (UV-Visible, IR, Raman, NMR), provide valuable data on bond lengths, vibrational frequencies, and electronic transitions, which are used to infer bond order.
Question 3: How does molecular orbital theory contribute to bond order determination?
Molecular orbital theory describes the electronic structure of molecules by constructing molecular orbitals from the linear combination of atomic orbitals. The energy levels and occupancy of these molecular orbitals provide insights into bond order and the strength of the chemical bond.
Question 4: What is the role of hybridization in bond order determination?
Hybridization involves the mixing of atomic orbitals to form new hybrid orbitals with different shapes and energies. The type of hybridization adopted by the bonding atoms determines the geometry and bond order of the molecule.
Question 5: How does resonance affect bond order?
Resonance occurs when multiple Lewis structures can be drawn for a molecule, indicating the delocalization of electrons. Resonance stabilizes the molecule and can influence bond order by altering the distribution of electrons in the bonding region.
Question 6: What are the applications of understanding bond order in B2?
Determining the bond order of B2 is crucial for predicting its chemical reactivity, designing new materials with specific properties, and understanding its role in various chemical processes.
These FAQs provide a concise overview of the key concepts related to finding bond order in B2. A deeper understanding of these concepts enables researchers to analyze and predict the properties and behavior of B2, opening up new avenues for scientific exploration and practical applications.
In the next section, we will delve into the advanced techniques and applications of bond order determination, exploring the frontiers of this field.
Tips for Determining the Bond Order of B2
This section provides practical tips to assist you in accurately determining the bond order of B2, ensuring reliable and meaningful results.
Tip 1: Utilize Molecular Orbital Theory
Analyze the molecular orbitals of B2 to determine the number of bonding and antibonding electrons, which directly influences the bond order.
Tip 2: Consider Hybridization
Identify the hybridization of the atomic orbitals involved in bonding, as it affects the geometry and bond order of the molecule.
Tip 3: Examine Bond Length
Measure the distance between the boron nuclei to obtain the bond length, which is inversely related to bond order, providing valuable insights.
Tip 4: Analyze Bond Energy
Determine the energy required to break the B-B bond, as bond energy is directly proportional to bond order, offering quantitative information.
Tip 5: Investigate Magnetic Properties
Examine the magnetic behavior of B2 to determine the presence or absence of unpaired electrons, which can provide clues about bond order.
Tip 6: Utilize Spectroscopic Data
Analyze spectroscopic data, such as UV-Visible and IR spectra, to obtain information about electronic transitions and vibrational frequencies, which can be correlated with bond order.
Tip 7: Employ Computational Chemistry
Utilize computational methods to solve the Schrdinger equation and calculate the electronic structure of B2, providing accurate estimates of bond order.
Tip 8: Consult Reference Materials
Refer to scientific literature, databases, and textbooks to gather additional insights, best practices, and experimental data related to bond order determination.
Understanding and applying these tips will enhance the accuracy and efficiency of your bond order determination, enabling a deeper understanding of the chemical properties and behavior of B2.
In the next section, we will explore advanced applications of bond order determination, showcasing its significance in various scientific disciplines and technological fields.
Conclusion
In this comprehensive exploration of “How to Find Bond Order of B2,” we have delved into the intricacies of determining the bond order of this diatomic molecule. Through the examination of key concepts such as molecular orbital theory, hybridization, bond length, and spectroscopic data, we have gained a deeper understanding of the electronic structure and bonding characteristics of B2.
The interconnectedness of these concepts highlights the importance of considering various factors when determining bond order. By combining experimental techniques with theoretical approaches, chemists can accurately predict the bond order of B2 and unravel its chemical properties and behavior. This understanding has far-reaching implications for fields such as materials science, catalysis, and nanotechnology.
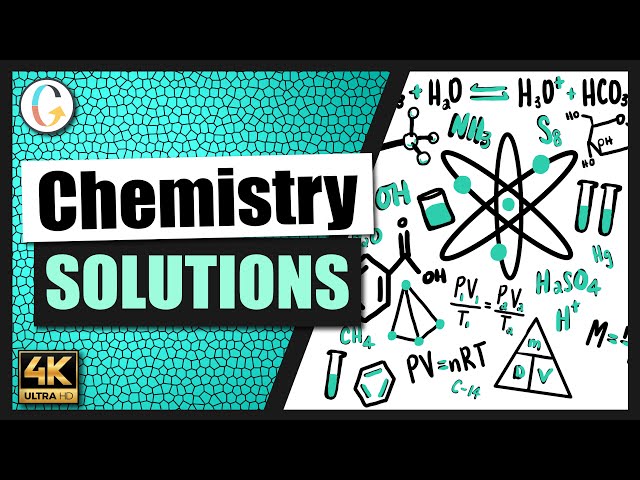