Determining the bond order of KO2 is essential for understanding the chemical structure and properties of this compound. The bond order, which represents the number of chemical bonds between two atoms, has important implications for KO2’s stability, reactivity, and electronic structure.
In chemistry, it is crucial to understand the bond order of molecules and ions to predict their chemical behavior, reactivity, and stability. Understanding the bond order of KO2 is particularly important for researchers in inorganic chemistry and materials science, as it provides insights into the bonding characteristics and electronic structure of this compound.
This article delves into the methods for calculating the bond order of KO2, exploring the underlying concepts and theoretical approaches. We will discuss the significance of bond order in understanding the chemical properties of KO2 and provide practical examples to illustrate the applications of this knowledge.
How to Find Bond Order of KO2
Determining the bond order of KO2 is crucial for understanding its chemical structure and properties. The bond order, a measure of the number of chemical bonds between two atoms, provides insights into KO2’s stability, reactivity, and electronic structure.
- Molecular Orbital Theory
- Valence Bond Theory
- Electronegativity
- Bond Length
- Bond Energy
- Oxidation State
- Resonance
- Hybridization
- Magnetic Properties
- Spectroscopic Data
Understanding these aspects helps us predict KO2’s chemical behavior, design new materials, and optimize its properties for various applications. By exploring the relationship between bond order and these factors, scientists can gain a deeper understanding of the electronic structure and reactivity of KO2.
Molecular Orbital Theory
Molecular Orbital Theory (MOT) provides the foundation for understanding the electronic structure and bonding in KO2. It describes the molecular orbitals as a combination of atomic orbitals, which can be used to determine the bond order and other properties of the molecule. According to MOT, the bond order is directly related to the number of electrons occupying the bonding molecular orbitals and inversely related to the number of electrons in the antibonding molecular orbitals. In the case of KO2, the bond order can be calculated using MOT by considering the molecular orbital configuration and the number of electrons in each orbital.
MOT is a critical component of understanding how to find the bond order of KO2 because it provides a theoretical framework for describing the electronic structure and bonding in the molecule. By applying MOT, we can determine the molecular orbital configuration, identify the bonding and antibonding orbitals, and calculate the bond order based on the electron distribution. This understanding is essential for predicting the stability, reactivity, and other properties of KO2.
Real-life examples of MOT being used to find the bond order of KO2 can be found in various research studies and applications. For instance, in materials science, MOT is used to design and optimize the properties of KO2-based materials, such as superconductors and catalysts. By understanding the bond order and electronic structure of KO2, scientists can tailor the material’s properties for specific applications.
In summary, MOT provides a powerful theoretical framework for understanding the electronic structure and bonding in KO2, which is essential for determining the bond order. By applying MOT, we can gain insights into the stability, reactivity, and other properties of KO2, enabling us to design and optimize materials for various applications.
Valence Bond Theory
Valence Bond Theory (VBT) provides a complementary approach to Molecular Orbital Theory for understanding the electronic structure and bonding in KO2. VBT describes the chemical bond as the overlap of atomic orbitals, resulting in the formation of localized electron pairs between the bonded atoms. The bond order in VBT is determined by the number of electron pairs shared between the atoms, which can be used to predict the stability and properties of the molecule.
VBT is a critical component of how to find the bond order of KO2 because it provides an intuitive and chemically meaningful way to describe the bonding in the molecule. By considering the overlap of atomic orbitals and the formation of electron pairs, we can determine the bond order and gain insights into the electronic structure and reactivity of KO2.
Real-life examples of VBT being used to find the bond order of KO2 can be found in various research studies and applications. For instance, in inorganic chemistry, VBT is used to understand the bonding and properties of transition metal complexes, such as KO2-based catalysts. By determining the bond order and electronic structure of KO2 in these complexes, scientists can design and optimize their catalytic activity for specific reactions.
In summary, VBT provides a valuable approach for understanding the electronic structure and bonding in KO2, which is essential for determining the bond order. By considering the overlap of atomic orbitals and the formation of electron pairs, VBT offers a chemically intuitive way to predict the stability and properties of KO2, enabling us to design and optimize materials for various applications.
Electronegativity
Electronegativity plays a significant role in understanding how to find the bond order of KO2. Electronegativity is a measure of the ability of an atom to attract electrons towards itself and is a critical factor in determining the type and strength of chemical bonds formed between atoms.
In the case of KO2, the electronegativity difference between potassium (K) and oxygen (O) influences the bond order. Oxygen is more electronegative than potassium, meaning it has a stronger attraction for electrons. This electronegativity difference results in the formation of a polar covalent bond between K and O, where the electron pair is shifted towards the oxygen atom. The polarity of the bond affects the bond order, as the electrons are not equally shared between the two atoms.
Real-life examples of electronegativity affecting the bond order of KO2 can be found in various research studies and applications. For instance, in materials science, understanding the electronegativity difference between K and O is crucial for designing and optimizing KO2-based materials, such as superconductors and batteries. By tailoring the electronegativity of the atoms involved, scientists can control the bond order and electronic properties of KO2, leading to improved performance and efficiency of these materials.
In summary, electronegativity is a key factor in determining the bond order of KO2. The electronegativity difference between potassium and oxygen influences the polarity of the bond, which in turn affects the electron distribution and bond order. Understanding the relationship between electronegativity and bond order is essential for predicting the stability, reactivity, and properties of KO2, enabling us to design and optimize materials for various applications.
Bond Length
Bond length is a crucial aspect in determining the bond order of KO2. It is a measure of the distance between the nuclei of the bonded atoms and provides insights into the strength and nature of the chemical bond.
- Atomic Radii: The atomic radii of potassium (K) and oxygen (O) influence the bond length in KO2. Larger atomic radii result in longer bond lengths due to the increased distance between the atomic nuclei.
- Bond Order: Bond length is inversely related to bond order. A higher bond order indicates a shorter bond length as the atoms are held more tightly together by stronger bonds.
- Electronegativity: The electronegativity difference between K and O affects the bond length. A greater electronegativity difference leads to a shorter bond length due to the stronger attraction of the more electronegative atom for electrons, pulling the atoms closer together.
- Hybridization: The hybridization of the atomic orbitals involved in bonding influences the bond length. Different hybridization states can result in varying bond lengths due to changes in the overlap and electron distribution between the orbitals.
Understanding the relationship between bond length and bond order is essential for predicting the stability, reactivity, and properties of KO2. By considering the factors that influence bond length, we can gain insights into the electronic structure and bonding characteristics of this compound.
Bond Energy
Bond energy, a measure of the strength of a chemical bond, plays a critical role in determining the bond order of KO2. The bond energy is directly related to the bond order, as a stronger bond corresponds to a higher bond energy and vice versa. This relationship stems from the fact that bond order represents the number of chemical bonds between two atoms, which in turn determines the strength of the bond and the amount of energy required to break it.
In the case of KO2, the bond energy between potassium (K) and oxygen (O) atoms is influenced by several factors, including the electronegativity difference, hybridization, and molecular orbital interactions. Understanding the bond energy is essential for predicting the stability, reactivity, and properties of KO2, as it provides insights into the strength and nature of the chemical bond.
Real-life examples of bond energy affecting the bond order of KO2 can be found in various research studies and applications. For instance, in materials science, understanding the bond energy of KO2 is crucial for designing and optimizing KO2-based materials, such as superconductors and batteries. By tailoring the bond energy through chemical modifications or doping, scientists can control the electronic properties and performance of these materials for specific applications.
In summary, bond energy is a critical component of understanding how to find the bond order of KO2. The relationship between bond energy and bond order provides insights into the strength and nature of the chemical bond, which is essential for predicting the stability, reactivity, and properties of KO2. This understanding enables the design and optimization of KO2-based materials for various technological applications.
Oxidation State
Oxidation state plays a crucial role in determining the bond order of KO2 as it provides insights into the distribution of electrons and the oxidation-reduction behavior of the atoms involved.
- Formal Charge: The formal charge of an atom in a molecule is calculated based on the electronegativity and bonding arrangements. It gives a theoretical estimate of the oxidation state of the atom.
- Average Oxidation State: In polyatomic ions or molecules with multiple metal atoms, the average oxidation state is determined by considering the overall charge and the oxidation states of other atoms.
- Real-life Examples: Oxidation state is essential in understanding the chemistry of transition metals, as it helps determine the electronic configuration and reactivity of metal complexes. In KO2, the oxidation state of potassium is +1, while that of oxygen is -2.
- Implications: The oxidation state provides valuable information about the bonding and properties of KO2. It helps predict the stability of the compound, its reactivity towards other species, and its potential applications in various chemical processes.
By understanding the oxidation states of potassium and oxygen in KO2, we can gain insights into the electron transfer and bonding interactions within the molecule. This understanding is crucial for determining the bond order and predicting the chemical behavior and properties of KO2.
Resonance
Resonance is a crucial concept in chemistry that provides a deeper understanding of the electronic structure and bonding in molecules like KO2. It involves the representation of a molecule as a hybrid of several contributing structures, known as resonance structures.
- Equivalent Resonance Structures: Resonance structures have the same arrangement of atoms but differ in the distribution of electrons. They contribute equally to the overall resonance hybrid.
- Delocalized Electrons: In resonance, certain electrons are not confined to a specific bond or atom but are delocalized over several atoms, forming a region of electron density.
- Real-Life Examples: Benzene is a classic example of resonance, where the six carbon atoms and six pi electrons are delocalized around the ring, contributing to its stability and unique chemical properties.
- Implications for Bond Order: Resonance can affect the bond order in KO2. By considering the different resonance structures and the delocalization of electrons, we can determine the average bond order and gain insights into the electronic structure and bonding interactions within the molecule.
In summary, resonance provides a comprehensive view of the electronic structure of KO2, enabling us to understand the delocalization of electrons, the equivalence of resonance structures, and their implications for determining the bond order. This concept is essential for accurately describing the bonding and properties of KO2 and similar molecules.
Hybridization
Hybridization is a crucial concept in understanding the electronic structure and bonding in KO2. It involves the mixing of atomic orbitals to form new hybrid orbitals with different shapes and energies. This hybridization plays a significant role in determining the bond order and properties of KO2.
- Types of Hybridization: In KO2, the potassium atom undergoes sp hybridization, where one s orbital and one p orbital combine to form two equivalent sp hybrid orbitals.
- Geometry and Bond Angles: The sp hybridization of potassium results in a linear geometry for KO2, with a bond angle of 180 degrees. This geometry is consistent with the observed bond length and bond strength of KO2.
- Bond Order: The sp hybridization of potassium and the overlap of the sp hybrid orbitals with the p orbitals of oxygen lead to the formation of a single sigma bond between potassium and oxygen. This results in a bond order of one in KO2.
- Stability and Reactivity: The hybridization in KO2 contributes to its stability and influences its reactivity. The linear geometry and strong sigma bond make KO2 a relatively stable compound. However, the presence of unhybridized p orbitals on oxygen allows for further bonding interactions, affecting the reactivity of KO2 in certain chemical reactions.
In summary, hybridization provides insights into the electronic structure and bonding in KO2. The sp hybridization of potassium, the resulting linear geometry, and the bond order of one are key factors in understanding the stability and reactivity of this compound. Hybridization is a fundamental concept that helps us determine the bond order and properties of KO2 and other molecules.
Magnetic Properties
Magnetic properties play a significant role in understanding how to find the bond order of KO2 and provide valuable insights into its electronic structure and bonding characteristics. The magnetic properties of a compound are influenced by the presence of unpaired electrons and their interactions, which can affect the overall bond order.
In the case of KO2, the potassium (K) atom has one valence electron, while each oxygen (O) atom has six valence electrons. The sp hybridization of the K atom results in one unpaired electron, making KO2 a paramagnetic compound. The presence of unpaired electrons leads to magnetic moments and interactions that contribute to the overall bond order of KO2.
Understanding the magnetic properties of KO2 is essential for determining its bond order accurately. Experimental techniques such as electron paramagnetic resonance (EPR) spectroscopy can be used to measure the magnetic properties and determine the number of unpaired electrons. This information, combined with other factors like electronegativity and hybridization, allows scientists to calculate the bond order of KO2 more precisely.
In summary, magnetic properties provide valuable information about the electronic structure and bonding in KO2. The presence of unpaired electrons and their interactions influence the magnetic properties, which can be measured experimentally and used to determine the bond order more accurately. Understanding the connection between magnetic properties and bond order is crucial for characterizing and predicting the behavior of KO2 in various chemical and physical processes.
Spectroscopic Data
Spectroscopic data plays a critical role in determining the bond order of KO2, providing valuable insights into its electronic structure and bonding characteristics. Various spectroscopic techniques, such as infrared (IR) spectroscopy and ultraviolet-visible (UV-Vis) spectroscopy, offer complementary information that aids in the accurate determination of bond order.
- Vibrational Spectroscopy: IR spectroscopy measures the vibrational frequencies of bonds, providing information about the bond strength and order. The stretching frequency of the K-O bond in KO2 can be used to estimate the bond order.
- Electronic Spectroscopy: UV-Vis spectroscopy probes the electronic transitions of molecules. The absorption maximum of KO2 in the UV-Vis spectrum corresponds to the electronic transition from the bonding to the antibonding molecular orbital, allowing for the calculation of the bond order.
- Magnetic Resonance Spectroscopy: Nuclear magnetic resonance (NMR) spectroscopy provides information about the local electronic environment around specific atoms. The chemical shift of the 39K nucleus in KO2 can be used to infer the electron density and bond order.
- Electron Paramagnetic Resonance Spectroscopy: Electron paramagnetic resonance (EPR) spectroscopy is used to study paramagnetic molecules with unpaired electrons. KO2 exhibits paramagnetism due to the presence of one unpaired electron, and EPR spectroscopy can provide insights into the electronic structure and bond order.
By combining the information obtained from various spectroscopic techniques, scientists can gain a comprehensive understanding of the electronic structure, bonding, and bond order of KO2. Spectroscopic data serves as a powerful tool for characterizing and predicting the behavior of KO2 in different chemical environments and applications.
Frequently Asked Questions (FAQs) about Finding Bond Order of KO2
This section addresses common questions and misconceptions about determining the bond order of KO2, providing clear and concise answers to enhance understanding.
Question 1: Why is determining the bond order of KO2 important?
Answer: Determining the bond order of KO2 provides crucial insights into its electronic structure, stability, and reactivity. It helps predict KO2’s chemical behavior, design materials, and optimize its properties for various applications.
Question 2: What are the common methods used to find the bond order of KO2?
Answer: Various methods are employed to find the bond order of KO2, including Molecular Orbital Theory, Valence Bond Theory, and experimental techniques like IR and UV-Vis spectroscopy.
Question 3: How does electronegativity affect the bond order of KO2?
Answer: Electronegativity influences the bond order by determining the distribution of electrons between potassium and oxygen atoms. A greater electronegativity difference leads to a higher bond order due to the stronger attraction of oxygen for electrons.
Question 4: What is the relationship between bond length and bond order in KO2?
Answer: Bond length and bond order are inversely related. A shorter bond length indicates a higher bond order, as the atoms are held together more strongly by stronger bonds.
Question 5: How does resonance affect the bond order of KO2?
Answer: Resonance can affect the bond order by delocalizing electrons over multiple atoms. This delocalization leads to an average bond order that better represents the electronic structure of KO2.
Question 6: What experimental techniques can be used to determine the bond order of KO2?
Answer: Experimental techniques like IR and UV-Vis spectroscopy, as well as magnetic resonance spectroscopy (EPR and NMR), provide valuable information about the electronic structure and bonding in KO2, aiding in the determination of bond order.
In summary, understanding the bond order of KO2 is crucial for unraveling its chemical properties and behavior. The various methods and factors discussed in these FAQs provide a comprehensive approach to accurately determining the bond order of KO2.
This knowledge serves as a foundation for further exploration of KO2’s applications in materials science, catalysis, and other fields.
Tips for Determining the Bond Order of KO2
To accurately determine the bond order of KO2, consider the following practical tips:
Tip 1: Understand the Basic Concepts: Begin by grasping the fundamental principles of bond order, including its definition, calculation methods, and significance in describing chemical bonding.
Tip 2: Utilize Molecular Orbital Theory and Valence Bond Theory: Apply both MOT and VBT approaches to gain a comprehensive understanding of the electronic structure and bonding in KO2.
Tip 3: Consider Electronegativity and Bond Properties: Analyze the electronegativity difference between potassium and oxygen to assess its impact on bond polarity, length, and strength.
Tip 4: Examine Resonance and Hybridization: Explore the effects of resonance and hybridization on the electronic structure and bond order of KO2.
Tip 5: Employ Spectroscopic Techniques: Utilize spectroscopic methods, such as IR and UV-Vis spectroscopy, to obtain experimental data that complements theoretical calculations.
Tip 6: Leverage Computational Chemistry Tools: Consider using computational chemistry software to model the electronic structure of KO2 and calculate its bond order accurately.
By following these tips, you can effectively determine the bond order of KO2, gaining valuable insights into its chemical bonding and properties. This understanding forms the basis for further exploration of KO2’s applications in catalysis, materials science, and other fields.
In the concluding section, we will delve into the practical implications of bond order determination, highlighting its significance in tailoring materials and optimizing chemical processes.
Conclusion
In summary, determining the bond order of KO2 provides valuable insights into its electronic structure, stability, and reactivity. By employing various theoretical and experimental approaches, we can accurately calculate the bond order and understand the nature of the chemical bond between potassium and oxygen in KO2.
This understanding has practical implications in fields such as materials science and catalysis. Tailoring the bond order of KO2 can lead to the development of materials with enhanced properties, such as superconductivity and improved catalytic activity. Furthermore, understanding the bond order allows us to optimize chemical processes involving KO2, leading to increased efficiency and selectivity.
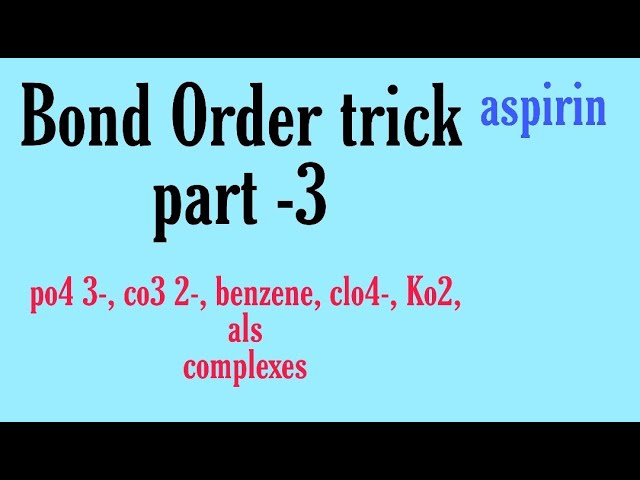