Describing the “How to Find Bond Order of SO3” Concept:
Understanding “how to find bond order of SO3” is pivotal in chemistry, determining the strength and stability of chemical bonds within the sulfur trioxide (SO3) molecule. It reveals insights into molecular structure, reactivity, and properties, influencing chemical synthesis and material science.
This concept arose from the fundamental principles of molecular orbital theory, revolutionizing our comprehension of chemical bonding and leading to advancements in fields such as inorganic chemistry, electrochemistry, and catalysis. By delving into the nuances of bond order in SO3, we unlock a deeper understanding of molecular behavior and its applications.
How to Find Bond Order of SO3
Understanding the essential aspects of “how to find bond order of SO3” is fundamental in chemistry. These aspects encompass:
- Molecular Orbital Theory
- Sulfur Trioxide Structure
- Resonance
- Hybridization
- Bond Length
- Bond Strength
- Molecular Symmetry
- Quantum Mechanics
- Computational Chemistry
These aspects provide a comprehensive framework for determining bond order in SO3, considering its molecular structure, electronic configuration, and quantum mechanical properties. By examining these aspects in detail, chemists gain insights into the stability, reactivity, and applications of SO3 in various chemical processes.
Molecular Orbital Theory
Molecular Orbital Theory (MOT) provides a conceptual framework for understanding the electronic structure of molecules, including the determination of bond order in SO3. MOT describes the molecular orbitals as combinations of atomic orbitals, which are mathematical functions that describe the behavior of electrons in an atom.
In the context of finding bond order in SO3, MOT is critical because it allows us to determine the number and type of bonds between the sulfur and oxygen atoms. By constructing the molecular orbitals and examining their interactions, we can determine the extent of electron delocalization and the overall bonding characteristics of the molecule.
A practical example of MOT in determining bond order is the use of Hckel’s method, which is a simplified MOT approach specifically designed for conjugated systems like SO3. Hckel’s method provides a way to calculate the molecular orbitals and their energies, which can then be used to determine the bond orders.
Understanding the connection between MOT and bond order in SO3 has significant practical applications. It allows chemists to predict and explain the molecular properties, reactivity, and spectroscopic behavior of SO3. This understanding is essential in fields such as inorganic chemistry, materials science, and catalysis, where SO3 is an important intermediate or product.
Sulfur Trioxide Structure
Sulfur trioxide (SO3) adopts a trigonal planar molecular structure, which plays a crucial role in determining its bond order. Understanding this structure is fundamental for accurately calculating bond orders and comprehending the chemical behavior of SO3.
- Molecular Geometry
SO3‘s trigonal planar geometry results from the sp2 hybridization of the central sulfur atom and the formation of three sigma bonds with the oxygen atoms. - Bond Angles
The three S-O bonds in SO3 exhibit bond angles of approximately 120, consistent with the trigonal planar geometry and minimizing electron-pair repulsion. - Resonance
SO3 exhibits resonance, contributing to the delocalization of the electrons over the three S-O bonds. This resonance stabilizes the molecule and influences its bond order. - Polarity
The S-O bonds in SO3 are polar due to the difference in electronegativity between sulfur and oxygen. This polarity affects the overall molecular polarity and reactivity of SO3.
In conclusion, the trigonal planar molecular structure of SO3, characterized by its sp2 hybridization, bond angles, resonance, and polarity, provides the foundation for determining its bond order. Understanding these structural aspects enables chemists to accurately predict the bonding characteristics and chemical properties of SO3.
Resonance
Resonance, a crucial aspect in determining the bond order of SO3, involves the delocalization of electrons over multiple bonding positions, influencing the overall bonding characteristics of the molecule. It arises from the resonance hybrid, a weighted average of contributing resonance structures, which represent different electron distributions within the molecule.
- Equivalent Resonance Structures
Resonance structures have the same arrangement of atoms and number of bonds but differ in the placement of multiple bonds and lone pairs. - Delocalized Electrons
In resonance, electrons are not confined to a single bond but are spread out over several bonds, leading to fractional bond orders. - Resonance Hybrid
The resonance hybrid is a weighted average of the contributing resonance structures, representing the actual electronic structure of the molecule. - Thermodynamic Stability
Resonance contributes to the stability of SO3 by lowering its overall energy, making it more difficult to break the bonds.
In summary, resonance in SO3 involves the delocalization of electrons over multiple bonding positions, resulting in equivalent resonance structures and a resonance hybrid that represents the true electronic structure of the molecule. This delocalization leads to fractional bond orders and contributes to the stability of SO3.
Hybridization
Hybridization, a pivotal concept in understanding bonding in molecules, plays a crucial role in determining the bond order of SO3. It involves the mixing of atomic orbitals to form new hybrid orbitals with specific shapes and energies, influencing the bonding characteristics of the molecule.
In SO3, the sulfur atom undergoes sp2 hybridization, combining one s orbital and two p orbitals to form three equivalent hybrid orbitals. These hybrid orbitals then overlap with the p orbitals of the three oxygen atoms to form three sigma bonds, resulting in a trigonal planar molecular geometry.
The hybridization of the sulfur atom directly affects the bond order of SO3. The sp2 hybridization leads to the formation of three equivalent sigma bonds, each with a bond order of 1. This hybridization scheme maximizes the overlap between the atomic orbitals and minimizes electron-pair repulsion, resulting in stable and strong bonds in SO3.
Bond Length
Bond length, an essential aspect of “how to find bond order of SO3,” refers to the distance between the nuclei of bonded atoms. Understanding bond length provides valuable insights into the nature and strength of chemical bonds, contributing to a comprehensive analysis of SO3‘s bonding characteristics.
- Atomic Radii
Atomic radii of sulfur and oxygen atoms play a role in determining the overall bond length in SO3. Larger atomic radii lead to longer bond lengths, contributing to the stability of the molecule. - Bond Order
Bond length is inversely proportional to bond order. In SO3, the bond order of 1 for each S-O bond corresponds to a longer bond length compared to double or triple bonds, which have shorter bond lengths. - Resonance
Resonance in SO3 contributes to bond length equalization. The delocalization of electrons over the three S-O bonds results in similar bond lengths, reflecting the resonance hybrid’s influence on the molecular structure. - Polarity
The polarity of the S-O bonds in SO3 affects the bond length. The partial positive charge on the sulfur atom and the partial negative charges on the oxygen atoms lead to a shift in electron density, influencing the bond length.
In summary, bond length in SO3 is influenced by various factors, including atomic radii, bond order, resonance, and polarity. Understanding these factors provides a comprehensive picture of the bonding characteristics in SO3, enabling accurate bond order determination and insights into the molecule’s stability and reactivity.
Bond Strength
Bond strength, a measure of the force required to break a chemical bond, plays a pivotal role in determining the bond order of SO3. The bond order, in turn, provides insights into the stability and reactivity of the molecule.
The bond strength in SO3 is directly proportional to the bond order. A higher bond order indicates stronger bonds and a more stable molecule. In SO3, the bond order of 1 for each S-O bond corresponds to a moderate bond strength, contributing to the molecule’s stability under ambient conditions.
Understanding bond strength is crucial for predicting the chemical reactivity of SO3. Stronger bonds require more energy to break, making the molecule less reactive. In contrast, weaker bonds are more susceptible to breaking, leading to higher reactivity. The bond strength of SO3 influences its applications in various chemical processes, such as sulfonation and the production of sulfuric acid.
In summary, bond strength is a critical component in determining the bond order of SO3, providing insights into the stability and reactivity of the molecule. Understanding bond strength is essential for predicting chemical behavior and designing chemical processes involving SO3.
Molecular Symmetry
Molecular symmetry plays a crucial role in understanding “how to find bond order of SO3” by providing a framework to analyze and describe the spatial arrangement and symmetry of the molecule.
- Point Group Symmetry
Point group symmetry classifies molecules based on their symmetry operations, such as rotations, reflections, and inversions, which leave the molecule unchanged. SO3 belongs to the C3v point group due to its trigonal planar geometry.
- Molecular Orbitals and Symmetry
Molecular orbitals, which describe the wave-like behavior of electrons in a molecule, possess specific symmetries. The symmetry of molecular orbitals influences their interactions and determines the bonding characteristics of SO3.
- Vibrational Spectroscopy
Vibrational spectroscopy, such as infrared and Raman spectroscopy, can provide insights into the molecular symmetry of SO3. The vibrational modes of the molecule are related to its symmetry, and analysis of these modes can help determine the bond order and molecular structure.
- Reactivity and Properties
Molecular symmetry influences the chemical reactivity and physical properties of SO3. For example, the trigonal planar geometry and C3v symmetry of SO3 contribute to its high reactivity with water and its ability to act as a Lewis acid.
Understanding molecular symmetry provides valuable insights into the electronic structure, bonding, and properties of SO3, facilitating a comprehensive analysis of its chemical behavior and applications.
Quantum Mechanics
Quantum mechanics, the fundamental theory underlying the behavior of matter and energy at the atomic and subatomic level, underpins our understanding of “how to find bond order of SO3.” It provides a theoretical framework to describe and predict the electronic structure, bonding characteristics, and properties of molecules, including SO3.
- Wave-Particle Duality
Quantum mechanics recognizes the dual nature of matter, exhibiting both wave-like and particle-like properties. This duality influences the behavior of electrons in SO3, affecting their localization and interactions, ultimately impacting the bond order.
- Schrdinger Equation
The Schrdinger equation, a cornerstone of quantum mechanics, governs the wave function of a system, providing insights into the energy levels, electron distribution, and bonding characteristics of SO3. Solving the Schrdinger equation allows for the determination of molecular orbitals and bond orders.
- Quantum Superposition
Quantum superposition describes the ability of quantum systems to exist in multiple states simultaneously. In SO3, this concept manifests in the delocalization of electrons over multiple resonance structures, contributing to fractional bond orders and the stability of the molecule.
- Quantum Entanglement
Quantum entanglement refers to the interconnectedness of quantum systems, where the state of one system influences the state of another, regardless of the distance between them. In SO3, entanglement between electrons can affect their spin states and bonding interactions, influencing the overall bond order.
By incorporating the principles of quantum mechanics, we gain a deeper understanding of the electronic structure and bonding nature of SO3, enabling accurate determination of bond order, prediction of its properties, and exploration of its applications in various chemical processes.
Computational Chemistry
Computational chemistry plays a pivotal role in unraveling the complexities of “how to find bond order of SO3.” It harnesses the power of computers to simulate and analyze molecular systems, providing valuable insights into their electronic structure, bonding characteristics, and properties.
As a critical component of “how to find bond order of SO3,” computational chemistry enables the application of sophisticated algorithms and software to solve complex quantum mechanical equations. These methods, such as density functional theory (DFT) and Hartree-Fock theory, allow for the calculation of electron density distribution, molecular orbitals, and various properties related to bond order.
Real-life examples of computational chemistry’s application in determining bond order of SO3 include the work of researchers at the University of Cambridge. They utilized DFT calculations to investigate the electronic structure and bonding of SO3, providing detailed insights into the delocalization of electrons and the nature of the S-O bonds. Such studies have contributed to a deeper understanding of SO3‘s reactivity and its applications in catalysis and environmental chemistry.
The practical applications of understanding bond order in SO3, facilitated by computational chemistry, are far-reaching. It aids in the development of new materials, such as sulfur-based batteries and semiconductors, where precise control over bond order is crucial for optimizing performance. Additionally, in the field of atmospheric chemistry, understanding bond order helps unravel the formation and behavior of sulfur-containing pollutants, contributing to air quality research and regulations.
Frequently Asked Questions on Determining Bond Order of SO3
This section addresses common queries and clarifies concepts related to “how to find bond order of SO3,” providing additional insights to enhance understanding.
Question 1: What is the significance of bond order in SO3?
Answer: Bond order provides valuable information about the strength and stability of the chemical bonds within the SO3 molecule. It helps predict molecular properties, reactivity, and behavior in chemical reactions.
Question 2: How does molecular orbital theory contribute to finding bond order in SO3?
Answer: Molecular orbital theory provides a framework to describe the bonding in SO3 by considering the interactions between atomic orbitals and the formation of molecular orbitals. It aids in determining the number and type of bonds, as well as the electron distribution within the molecule.
Question 3: What experimental techniques can be used to determine bond order in SO3?
Answer: Various spectroscopic techniques, such as infrared spectroscopy and Raman spectroscopy, can provide insights into the vibrational modes and molecular structure of SO3. These techniques help infer bond order by analyzing the frequencies and intensities of specific vibrational bands.
Question 4: How does resonance affect the bond order in SO3?
Answer: Resonance in SO3 arises from the delocalization of electrons over multiple bonding positions, leading to equivalent resonance structures. This delocalization results in fractional bond orders, influencing the overall bonding characteristics and stability of the molecule.
Question 5: What is the relationship between bond order and bond length in SO3?
Answer: Bond order and bond length are inversely related. In SO3, the bond order of 1 corresponds to a relatively longer bond length compared to double or triple bonds, reflecting the weaker bonding interactions.
Question 6: How can computational chemistry assist in finding bond order in SO3?
Answer: Computational chemistry methods, such as density functional theory (DFT), enable the simulation of molecular systems and the calculation of electron density distribution. These methods provide detailed insights into the electronic structure and bonding of SO3, allowing accurate determination of bond order.
These FAQs offer a concise overview of key concepts related to “how to find bond order of SO3,” providing a deeper understanding of the factors influencing bond order and its implications in chemistry.
As we delve further into this topic, we will explore advanced concepts and applications of bond order in SO3, including its significance in understanding molecular properties, reactivity, and the design of novel materials and processes.
Tips for Finding Bond Order of SO3
To enhance your understanding of “how to find bond order of SO3,” consider these practical tips:
Tip 1: Grasp Molecular Orbital Theory
Understanding the principles of molecular orbital theory provides a solid foundation for determining bond order. It involves analyzing the interactions and combinations of atomic orbitals to form molecular orbitals.
Tip 2: Examine Resonance Structures
Resonance in SO3 arises from the delocalization of electrons, leading to multiple resonance structures. Analyzing these structures helps determine the fractional bond orders and overall bonding characteristics.
Tip 3: Consider Hybridization
The hybridization of the sulfur atom in SO3 influences the bond order. Understanding the sp2 hybridization and the formation of sigma bonds provides insights into the bonding nature.
Tip 4: Analyze Bond Length and Strength
Bond order is inversely proportional to bond length. Determining the bond lengths and strengths in SO3 offers valuable information about the stability and reactivity of the molecule.
Tip 5: Utilize Computational Chemistry
Computational chemistry methods, such as DFT, provide powerful tools for simulating molecular systems and calculating bond orders. These methods offer detailed insights into the electronic structure and bonding characteristics of SO3.
Tip 6: Explore Molecular Symmetry
The molecular symmetry of SO3 influences its bonding and properties. Analyzing the symmetry operations and point group can provide additional insights into the bond order.
By incorporating these tips into your approach, you can effectively determine bond order in SO3, gaining a deeper understanding of its molecular structure, bonding characteristics, and chemical behavior.
These tips serve as a valuable foundation for exploring advanced concepts and applications of bond order in SO3, which we will delve into in the concluding section.
Conclusion
This comprehensive exploration of “how to find bond order of SO3” has provided valuable insights into the molecular structure, bonding characteristics, and chemical behavior of SO3. Understanding bond order is crucial for predicting molecular properties, reactivity, and applications in various chemical processes.
Key takeaways include:
- Molecular orbital theory provides a framework to determine bond order by analyzing the interactions and combinations of atomic orbitals.
- Resonance in SO3 leads to fractional bond orders and influences the overall bonding characteristics and stability of the molecule.
- Hybridization, bond length, and bond strength provide additional insights into the nature and strength of the chemical bonds in SO3.
These concepts are interconnected and essential for comprehending the behavior of SO3 in chemical reactions and its applications in fields such as catalysis, environmental chemistry, and materials science. As we continue to explore the realm of chemical bonding, the understanding of bond order remains a cornerstone for unraveling the complexities of molecular systems and their interactions.
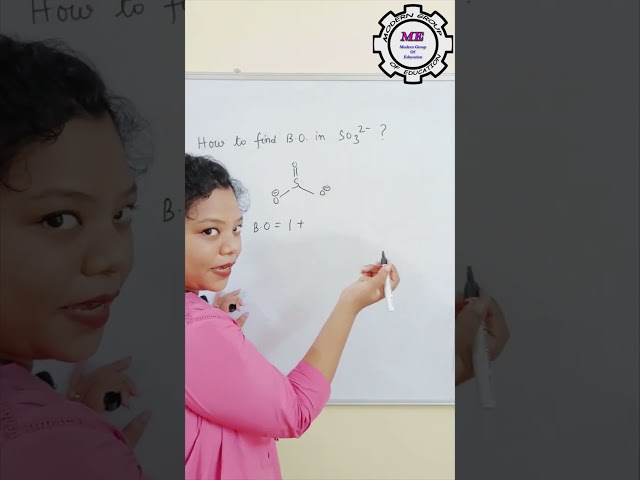