How to find bond order for resonance structures refers to a crucial concept in chemistry that involves determining the overall bond order within a molecule that possesses multiple resonance structures. Resonance structures are different representations of the same molecule that show the delocalization of electrons across multiple atoms. These structures are essential for understanding the electronic structure and properties of molecules.
The bond order is a measure of the strength and nature of the chemical bond between two atoms. Finding the bond order for resonance structures is key to predicting the stability, reactivity, and other physical properties of a compound. Historically, the concept of resonance was first introduced by Linus Pauling in 1928, revolutionizing the field of chemistry.
This article will delve into the methods and significance of finding bond order for resonance structures, exploring its applications in various chemical contexts and providing a comprehensive understanding of this fundamental concept.
How to Find Bond Order for Resonance Structures
Finding the bond order for resonance structures is a crucial aspect of chemistry, providing insights into the electronic structure and properties of molecules. Key aspects to consider include:
- Resonance contributors
- Delocalization
- Bond length
- Bond strength
- Molecular stability
- Reactivity
- Quantum mechanics
- Hybridization
- Molecular orbitals
- Electron density
Understanding these aspects allows chemists to predict the behavior and properties of compounds, design new materials, and explore chemical reactions. By considering resonance structures and bond order, chemists can gain a deeper understanding of the molecular world.
Resonance contributors
Resonance contributors are different Lewis structures that represent the same molecule. They are used to describe the delocalization of electrons across multiple atoms, which results in the formation of resonance hybrids. Resonance contributors are crucial for understanding how to find bond order for resonance structures.
The bond order is a measure of the strength and nature of the chemical bond between two atoms. It can be determined by considering the number of bonds between the atoms, as well as the number of electrons that are delocalized across the bonds. In the case of resonance structures, the bond order is an average of the bond orders in the individual resonance contributors.
For example, benzene is a molecule that has six resonance contributors. Each resonance contributor shows a different arrangement of double bonds and single bonds between the carbon atoms. The bond order between any two carbon atoms in benzene is 1.5, which means that the bond is stronger than a single bond but weaker than a double bond. This is because the electrons in the benzene ring are delocalized across all six carbon atoms, resulting in an equal distribution of bond strength.
Understanding the concept of resonance contributors and their connection to bond order is essential for predicting the behavior and properties of molecules. It allows chemists to design new materials, understand chemical reactions, and develop new drugs and treatments.
Delocalization
Delocalization plays a crucial role in determining the bond order for resonance structures. It refers to the distribution of electrons over several atoms, rather than being confined to a specific pair of atoms as in a localized bond. This concept significantly influences the strength and properties of bonds within a molecule.
- Electron Delocalization: Electrons are not restricted to individual bonds but can occupy molecular orbitals that encompass multiple atomic centers, leading to delocalized electron density and influencing bond order calculations.
- Resonance Hybrids: Delocalized electrons contribute to the formation of resonance hybrids, which are combinations of resonance structures that represent the average electronic structure of the molecule. Bond order is determined by considering the contributions from each resonance structure.
- Bond Order and Delocalization: Delocalization reduces the bond order between specific pairs of atoms compared to localized bonds. This is because the electrons are not exclusively shared between two atoms but are distributed over a larger region.
- Aromatic Systems: Delocalization is particularly evident in aromatic compounds, where resonance structures contribute equally to the overall electronic structure. The delocalized electrons result in increased stability and unique properties, such as in benzene.
Understanding delocalization is essential for accurately finding bond order in resonance structures. It provides insights into the electronic structure, stability, and reactivity of molecules, enabling chemists to predict and design materials with specific properties.
Bond length
Bond length, a crucial aspect of finding bond order for resonance structures, provides insights into the strength and nature of chemical bonds. It refers to the equilibrium distance between the nuclei of two bonded atoms.
- Bond Order and Length: Bond order and bond length are inversely related. Stronger bonds, characterized by higher bond order, exhibit shorter bond lengths due to increased electron sharing and stronger attractive forces.
- Resonance and Bond Length: Resonance, involving the delocalization of electrons across multiple atoms, affects bond lengths. Delocalized electrons reduce the bond order between specific pairs of atoms, leading to longer bond lengths compared to localized bonds.
- Experimental Determination: Bond lengths can be experimentally determined using techniques like X-ray crystallography and microwave spectroscopy. These techniques provide precise measurements of internuclear distances, contributing to accurate bond order calculations.
- Predicting Bond Length: Theoretical methods, such as quantum chemistry calculations, can predict bond lengths based on the electronic structure and molecular geometry. These predictions aid in understanding bond order and the stability of resonance structures.
Understanding bond length within the context of resonance structures is crucial for comprehending molecular properties and predicting reactivity. It provides valuable insights into the nature of chemical bonds, allowing chemists to design and synthesize materials with desired characteristics.
Bond Strength
Within the context of “how to find bond order for resonance structures,” bond strength plays a pivotal role. It encompasses the energetic favorability and stability of chemical bonds, providing insights into molecular structure and reactivity.
- Bond Dissociation Energy: The energy required to break a bond, quantifying the strength of the bond. Higher bond dissociation energies indicate stronger bonds.
- Bond Order: Bond order and bond strength are directly proportional. Resonance structures with higher bond orders exhibit stronger bonds due to increased electron sharing.
- Delocalization: Delocalized electrons in resonance structures reduce bond strength compared to localized bonds. Delocalization weakens bonds by distributing electron density over multiple atoms.
- Hybridization: The hybridization of atomic orbitals influences bond strength. For instance, sp3 hybridized bonds are typically stronger than sp2 hybridized bonds due to better orbital overlap.
Understanding bond strength in resonance structures aids in predicting molecular stability, reactivity patterns, and physical properties. It enables chemists to design molecules with tailored bond strengths for specific applications, ranging from pharmaceuticals to advanced materials.
Molecular stability
In the context of “how to find bond order for resonance structures”, molecular stability plays a crucial role in determining the overall stability and properties of the molecule. It encompasses various aspects that influence the molecule’s resistance to change or decomposition.
- Thermodynamic Stability: Refers to the molecule’s energy state, where lower energy states correspond to higher stability. Resonance structures contribute to stability by distributing the electron density and lowering the overall energy.
- Kinetic Stability: Relates to the molecule’s resistance to chemical reactions and decomposition pathways. Resonance structures can hinder reactions by providing alternative resonance forms that increase the activation energy required for bond breaking.
- Structural Stability: Describes the molecule’s resistance to changes in its geometry or conformation. Resonance structures can influence molecular shape and stability by delocalizing electrons and strengthening certain bonds.
Understanding molecular stability in the context of resonance structures enables chemists to predict the behavior and reactivity of molecules, design new materials with desired properties, and develop strategies for stabilizing molecules in various environments.
Reactivity
In the context of “how to find bond order for resonance structures,” reactivity refers to the propensity of a molecule to undergo chemical reactions. It encompasses various aspects that influence the molecule’s behavior and interactions with other species.
- Kinetic Reactivity: Relates to the reaction rate and activation energy of a molecule. Resonance structures can affect kinetic reactivity by influencing the distribution of electron density and the stability of transition states.
- Thermodynamic Reactivity: Describes the favorability of a reaction based on enthalpy and entropy changes. Resonance structures can contribute to thermodynamic reactivity by stabilizing products or intermediates.
- Regioselectivity: Involves the preference for a reaction to occur at a specific site within a molecule. Resonance structures can influence regioselectivity by directing incoming reactants to more reactive positions.
- Stereoselectivity: Relates to the control of stereochemistry in a reaction. Resonance structures can impact stereoselectivity by stabilizing specific stereoisomers or transition states.
Understanding reactivity in relation to resonance structures enables chemists to predict reaction outcomes, design synthetic strategies, and develop new molecules with tailored reactivity profiles.
Quantum mechanics
Quantum mechanics is a fundamental theory in physics that provides a framework to describe and predict the behavior of matter at the atomic and subatomic levels. It plays a crucial role in understanding “how to find bond order for resonance structures” as it offers a theoretical foundation for the electronic structure and bonding in molecules.
- Wave-particle duality: Matter exhibits both wave-like and particle-like properties. Electrons, for instance, can behave like waves, allowing them to spread out and occupy multiple positions simultaneously, which is essential for understanding resonance and delocalization.
- Superposition: Quantum systems can exist in multiple states or positions simultaneously until measured or observed. This concept helps explain the resonance phenomenon, where a molecule can adopt different resonance structures that contribute to the overall electronic structure.
- Quantization: Energy levels in atoms and molecules are discrete, meaning they can only exist in specific, quantized states. This principle underlies the formation of molecular orbitals and the distribution of electrons within resonance structures.
- Uncertainty principle: The position and momentum of a particle cannot be known with absolute certainty simultaneously. This uncertainty has implications for determining precise bond orders in resonance structures, as it limits the ability to know the exact location of electrons.
These fundamental aspects of quantum mechanics provide a theoretical framework for understanding and predicting the electronic structure and bonding in resonance structures. It enables chemists to gain insights into molecular properties, reactivity, and behavior at the quantum level.
Hybridization
In the context of “how to find bond order for resonance structures,” hybridization is crucial for understanding the electronic structure and bonding within molecules. By considering the hybridization of atomic orbitals, chemists can determine the geometry and properties of resonance structures, which in turn influences bond order calculations.
- sp3 Hybridization: Occurs when an atomic orbital hybridizes with three p orbitals, resulting in four equivalent hybrid orbitals directed towards the corners of a tetrahedron. This hybridization is common in molecules with single bonds, such as methane (CH4).
- sp2 Hybridization: Involves the hybridization of an atomic orbital with two p orbitals, forming three equivalent hybrid orbitals arranged in a trigonal planar geometry. This hybridization is found in molecules with double bonds, such as ethene (C2H4).
- sp Hybridization: Occurs when an atomic orbital hybridizes with one p orbital, resulting in two equivalent hybrid orbitals oriented 180 degrees apart. This hybridization is observed in molecules with triple bonds, such as acetylene (C2H2).
Understanding hybridization enables chemists to determine the molecular geometry and bond angles, which are crucial factors in finding bond order for resonance structures. By considering the hybridization of atomic orbitals, chemists can accurately predict the electronic structure and properties of molecules, aiding in the design and synthesis of new materials with tailored properties.
Molecular orbitals
In the context of “how to find bond order for resonance structures,” molecular orbitals play a fundamental role in determining the electronic structure and bonding within molecules. Molecular orbitals are mathematical functions that describe the wave-like behavior of electrons within a molecule, providing insights into their distribution and interactions.
To find bond order for resonance structures, it is crucial to consider the molecular orbitals involved in the resonance. Resonance structures are different Lewis structures that represent the same molecule, and their resonance hybrid is a combination of these structures. The molecular orbitals of the resonance hybrid represent the average electronic structure of the molecule, and their energies and occupations determine the bond order.
Real-life examples of molecular orbitals in “how to find bond order for resonance structures” include benzene and ethene. Benzene is an aromatic compound with a resonance structure involving six equivalent resonance forms. The molecular orbitals of benzene are delocalized over the entire ring, resulting in an equal distribution of bond order between the carbon atoms.
Understanding the connection between molecular orbitals and bond order for resonance structures has practical applications in chemistry. It enables the prediction of molecular properties, such as stability, reactivity, and magnetic behavior. This understanding is also essential for designing and synthesizing new materials with tailored electronic and structural properties.
Electron density
Electron density, a fundamental concept in chemistry, plays a significant role in determining bond order for resonance structures. It refers to the probability of finding an electron in a particular region of space around an atom or molecule.
In the context of resonance structures, electron density is crucial for understanding the delocalization of electrons. Resonance structures are different Lewis structures that represent the same molecule, and they contribute to the resonance hybrid, which is a combination of these structures. The electron density in the resonance hybrid is distributed over the entire molecule, rather than being localized between specific atoms.
Real-life examples of electron density in “how to find bond order for resonance structures” include benzene and ethene. Benzene is an aromatic compound with a resonance structure involving six equivalent resonance forms. The electron density in benzene is delocalized over the entire ring, resulting in an equal distribution of bond order between the carbon atoms.
Understanding the connection between electron density and bond order for resonance structures has practical applications in chemistry. It enables the prediction of molecular properties, such as stability, reactivity, and magnetic behavior. This understanding is also essential for designing and synthesizing new materials with tailored electronic and structural properties.
Frequently Asked Questions on How to Find Bond Order for Resonance Structures
This section addresses common queries and misconceptions regarding the determination of bond order in resonance structures.
Question 1: What is the significance of resonance structures in finding bond order?
Answer: Resonance structures are different Lewis structures representing the same molecule. They contribute to the resonance hybrid, whose electron density is delocalized over the molecule, influencing bond order calculations.
Question 2: How do you determine the bond order for a particular bond in a resonance structure?
Answer: The bond order is calculated as the average of bond orders in the contributing resonance structures, considering the number of bonds and delocalized electrons between the atoms.
Question 3: Can resonance structures have different bond orders for the same bond?
Answer: Yes, resonance structures can show different bond orders for the same bond due to the varying distribution of delocalized electrons in each structure.
Question 4: How does hybridization affect bond order in resonance structures?
Answer: Hybridization influences the geometry and overlap of atomic orbitals, which can impact the delocalization of electrons and, consequently, the bond order in resonance structures.
Question 5: What is the relationship between bond order and molecular stability?
Answer: Bond order is directly related to molecular stability. Higher bond orders indicate stronger bonds, leading to more stable resonance structures and lower overall molecular energy.
Question 6: How can experimental techniques help determine bond order in resonance structures?
Answer: Techniques like X-ray crystallography and spectroscopy provide experimental evidence of bond lengths and electron density distribution, which can be used to infer bond order in resonance structures.
These FAQs provide fundamental insights into finding bond order for resonance structures, highlighting the significance of electron delocalization, hybridization, and experimental techniques in understanding molecular structure and bonding.
In the next section, we will delve into advanced applications of bond order determination in resonance structures, exploring their implications for chemical reactivity, molecular design, and materials science.
Tips for Finding Bond Order in Resonance Structures
To effectively determine bond order in resonance structures, consider the following practical tips:
Tip 1: Identify resonance contributors: Recognize and analyze the different Lewis structures that contribute to the resonance hybrid.
Tip 2: Delocalize electrons: Understand how electrons are distributed over multiple atoms in resonance structures, leading to delocalization.
Tip 3: Calculate bond order: Determine the bond order for each bond by considering the number of bonds and delocalized electrons between the atoms.
Tip 4: Consider hybridization: Take into account the hybridization of atomic orbitals, as it influences electron distribution and bond order.
Tip 5: Examine molecular geometry: Analyze the molecular geometry to understand the spatial arrangement of atoms and the impact on bond order.
Tip 6: Utilize molecular orbital theory: Apply molecular orbital theory to describe electron behavior and interactions, providing insights into bond order.
Tip 7: Employ computational methods: Use computational chemistry techniques to calculate bond orders accurately and efficiently.
Tip 8: Validate results experimentally: Consider experimental techniques like X-ray crystallography and spectroscopy to confirm bond order determinations.
By implementing these tips, you can enhance your understanding of bond order in resonance structures and gain a deeper grasp of molecular electronic structures.
In the next section, we will explore advanced applications of bond order determination in resonance structures, uncovering their significance in chemical reactivity, molecular design, and materials science.
Conclusion
This comprehensive exploration of “how to find bond order for resonance structures” has provided valuable insights into the electronic structure and bonding within molecules. Key concepts such as resonance, delocalization, hybridization, and molecular orbital theory have been examined, emphasizing their interconnections and significance in bond order determination.
The ability to find bond order for resonance structures empowers chemists to understand and predict molecular properties, reactivity, and stability. This knowledge has far-reaching applications in fields like organic chemistry, materials science, and biochemistry. It enables the design of new materials with tailored properties, the optimization of chemical reactions, and the development of drugs and therapeutic agents.
As we continue to delve deeper into the realm of chemical bonding and molecular behavior, the exploration of resonance structures and bond order determination will remain a cornerstone of our understanding. It is through this ongoing pursuit of knowledge that we unlock the potential to shape the molecular world and address the challenges of the 21st century.
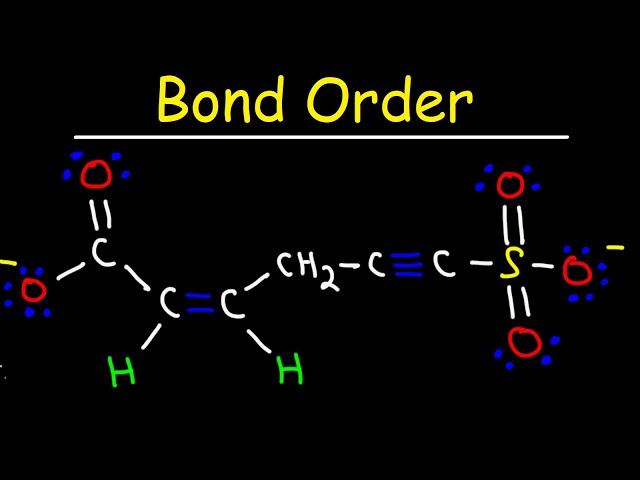